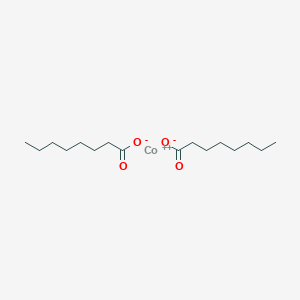
Cobalt dioctanoate
- Click on QUICK INQUIRY to receive a quote from our team of experts.
- With the quality product at a COMPETITIVE price, you can focus more on your research.
Overview
Description
Cobalt dioctanoate is a chemical compound that has been extensively studied in the field of chemistry. It is a cobalt-based catalyst that is commonly used in organic synthesis reactions. Cobalt dioctanoate is a versatile catalyst that has been used in various chemical reactions such as polymerization, oxidation, and esterification. The compound is known for its efficiency, effectiveness, and cost-effectiveness, which makes it an attractive option for many researchers.
Mechanism of Action
The mechanism of action of cobalt dioctanoate involves the coordination of the cobalt ion with the reactants. The compound acts as a Lewis acid catalyst, which means that it accepts electron pairs from the reactants. This results in the formation of a complex between the cobalt ion and the reactants. The complex then undergoes various chemical reactions, resulting in the formation of the desired product.
Biochemical and Physiological Effects:
Cobalt dioctanoate is not commonly used in biochemical and physiological studies. However, studies have shown that exposure to cobalt compounds can result in various health effects such as respiratory problems, skin irritation, and allergic reactions. Therefore, it is important to handle cobalt dioctanoate with care and follow proper safety protocols.
Advantages and Limitations for Lab Experiments
The advantages of using cobalt dioctanoate as a catalyst in lab experiments include its efficiency, effectiveness, and cost-effectiveness. The compound is readily available and can be easily synthesized in the lab. However, the limitations of using cobalt dioctanoate include its toxicity and potential health effects. Therefore, it is important to handle the compound with care and follow proper safety protocols.
Future Directions
There are several future directions for research on cobalt dioctanoate. One possible direction is the development of new and improved synthesis methods for the compound. Another direction is the exploration of new applications for cobalt dioctanoate in various chemical reactions. Additionally, research can be conducted to investigate the potential health effects of cobalt dioctanoate and to develop proper safety protocols for handling the compound.
Synthesis Methods
The synthesis of cobalt dioctanoate involves the reaction between cobalt acetate and octanoic acid. The reaction is carried out in the presence of a solvent such as toluene or xylene. The mixture is heated to a temperature of about 120°C to 150°C for several hours until the reaction is complete. The resulting product is a dark green liquid that is soluble in organic solvents.
Scientific Research Applications
Cobalt dioctanoate has been extensively studied in the field of organic synthesis. It is commonly used as a catalyst in various chemical reactions such as polymerization, oxidation, and esterification. The compound has been used in the synthesis of various organic compounds such as polyesters, polyamides, and polyurethanes. Cobalt dioctanoate has also been used in the production of various industrial products such as paints, coatings, and adhesives.
properties
CAS RN |
1588-79-0 |
---|---|
Product Name |
Cobalt dioctanoate |
Molecular Formula |
C16H30CoO4 |
Molecular Weight |
345.34 g/mol |
IUPAC Name |
cobalt(2+);octanoate |
InChI |
InChI=1S/2C8H16O2.Co/c2*1-2-3-4-5-6-7-8(9)10;/h2*2-7H2,1H3,(H,9,10);/q;;+2/p-2 |
InChI Key |
HWVKIRQMNIWOLT-UHFFFAOYSA-L |
SMILES |
CCCCCCCC(=O)[O-].CCCCCCCC(=O)[O-].[Co+2] |
Canonical SMILES |
CCCCCCCC(=O)[O-].CCCCCCCC(=O)[O-].[Co+2] |
Other CAS RN |
6700-85-2 1588-79-0 |
Pictograms |
Irritant; Environmental Hazard |
Origin of Product |
United States |
Disclaimer and Information on In-Vitro Research Products
Please be aware that all articles and product information presented on BenchChem are intended solely for informational purposes. The products available for purchase on BenchChem are specifically designed for in-vitro studies, which are conducted outside of living organisms. In-vitro studies, derived from the Latin term "in glass," involve experiments performed in controlled laboratory settings using cells or tissues. It is important to note that these products are not categorized as medicines or drugs, and they have not received approval from the FDA for the prevention, treatment, or cure of any medical condition, ailment, or disease. We must emphasize that any form of bodily introduction of these products into humans or animals is strictly prohibited by law. It is essential to adhere to these guidelines to ensure compliance with legal and ethical standards in research and experimentation.