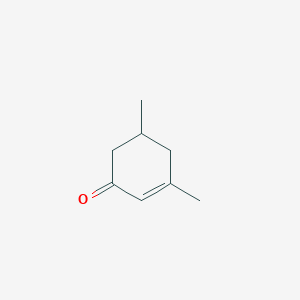
3,5-Dimethyl-2-cyclohexen-1-one
Overview
Description
3,5-Dimethyl-2-cyclohexen-1-one is an organic compound with the molecular formula C8H12O. It is a cyclic ketone, specifically a cyclohexenone, characterized by the presence of two methyl groups at the 3 and 5 positions on the cyclohexene ring. This compound is known for its versatility in organic synthesis and is used as a building block for various chemical products.
Mechanism of Action
Target of Action
3,5-Dimethyl-2-cyclohexen-1-one is a versatile electrophile . It primarily targets organocopper nucleophiles, enol silanes, and phosphoniosilylations . These targets play a crucial role in various addition reactions.
Mode of Action
The compound interacts with its targets through a series of addition reactions . It acts as an electrophile, attracting electron-rich nucleophiles. This interaction results in the formation of new covalent bonds, leading to the creation of complex organic structures.
Biochemical Pathways
It is known to participate in conjugate addition reactions with organocopper nucleophiles, michael reactions with enol silanes, and phosphoniosilylations . These reactions can lead to various downstream effects, including the synthesis of complex organic compounds.
Preparation Methods
Synthetic Routes and Reaction Conditions
3,5-Dimethyl-2-cyclohexen-1-one can be synthesized through several methods, including:
Robinson Annulation: This method involves the reaction of methyl vinyl ketone with a diketone in the presence of a base, leading to the formation of the cyclohexenone ring.
Claisen-Schmidt Condensation: This involves the reaction between 2,4-pentanedione and cyclohexanone with a base catalyst.
Aldol Condensation: This method involves the reaction of an aldehyde with a ketone in the presence of a base to form a β-hydroxy ketone, which is then dehydrated to form the cyclohexenone.
Industrial Production Methods
Industrial production of this compound typically involves large-scale Claisen-Schmidt condensation due to its efficiency and high yield. The reaction is carried out under controlled conditions to ensure the purity and quality of the final product.
Chemical Reactions Analysis
Types of Reactions
3,5-Dimethyl-2-cyclohexen-1-one undergoes various chemical reactions, including:
Oxidation: The compound can be oxidized to form corresponding carboxylic acids or ketones.
Reduction: Reduction of this compound can yield cyclohexanol derivatives.
Substitution: The compound can undergo nucleophilic substitution reactions, particularly at the carbonyl carbon.
Common Reagents and Conditions
Oxidation: Common oxidizing agents include potassium permanganate and chromium trioxide.
Reduction: Reducing agents such as sodium borohydride and lithium aluminum hydride are commonly used.
Substitution: Nucleophiles such as Grignard reagents and organolithium compounds are used for substitution reactions.
Major Products Formed
Oxidation: Carboxylic acids and ketones.
Reduction: Cyclohexanol derivatives.
Substitution: Various substituted cyclohexenones depending on the nucleophile used.
Scientific Research Applications
3,5-Dimethyl-2-cyclohexen-1-one has a wide range of applications in scientific research:
Chemistry: It is used as a building block for the synthesis of complex organic molecules.
Biology: The compound is used in the synthesis of biologically active molecules and pharmaceuticals.
Medicine: It serves as an intermediate in the production of various drugs.
Industry: The compound is used in the manufacture of fragrances, flavors, and other fine chemicals.
Comparison with Similar Compounds
3,5-Dimethyl-2-cyclohexen-1-one can be compared with other similar compounds such as:
2-Cyclohexen-1-one: Lacks the methyl groups at the 3 and 5 positions, making it less sterically hindered.
3-Methyl-2-cyclohexenone: Contains only one methyl group, resulting in different reactivity and properties.
4,4-Dimethylcyclohexanone: Has methyl groups at different positions, leading to different chemical behavior.
The uniqueness of this compound lies in its specific substitution pattern, which influences its reactivity and applications in organic synthesis.
Properties
IUPAC Name |
3,5-dimethylcyclohex-2-en-1-one | |
---|---|---|
Source | PubChem | |
URL | https://pubchem.ncbi.nlm.nih.gov | |
Description | Data deposited in or computed by PubChem | |
InChI |
InChI=1S/C8H12O/c1-6-3-7(2)5-8(9)4-6/h4,7H,3,5H2,1-2H3 | |
Source | PubChem | |
URL | https://pubchem.ncbi.nlm.nih.gov | |
Description | Data deposited in or computed by PubChem | |
InChI Key |
NOQKKFBBAODEHN-UHFFFAOYSA-N | |
Source | PubChem | |
URL | https://pubchem.ncbi.nlm.nih.gov | |
Description | Data deposited in or computed by PubChem | |
Canonical SMILES |
CC1CC(=CC(=O)C1)C | |
Source | PubChem | |
URL | https://pubchem.ncbi.nlm.nih.gov | |
Description | Data deposited in or computed by PubChem | |
Molecular Formula |
C8H12O | |
Source | PubChem | |
URL | https://pubchem.ncbi.nlm.nih.gov | |
Description | Data deposited in or computed by PubChem | |
DSSTOX Substance ID |
DTXSID20870848 | |
Record name | 3,5-Dimethylcyclohex-2-en-1-one | |
Source | EPA DSSTox | |
URL | https://comptox.epa.gov/dashboard/DTXSID20870848 | |
Description | DSSTox provides a high quality public chemistry resource for supporting improved predictive toxicology. | |
Molecular Weight |
124.18 g/mol | |
Source | PubChem | |
URL | https://pubchem.ncbi.nlm.nih.gov | |
Description | Data deposited in or computed by PubChem | |
CAS No. |
1123-09-7 | |
Record name | 3,5-Dimethyl-2-cyclohexen-1-one | |
Source | CAS Common Chemistry | |
URL | https://commonchemistry.cas.org/detail?cas_rn=1123-09-7 | |
Description | CAS Common Chemistry is an open community resource for accessing chemical information. Nearly 500,000 chemical substances from CAS REGISTRY cover areas of community interest, including common and frequently regulated chemicals, and those relevant to high school and undergraduate chemistry classes. This chemical information, curated by our expert scientists, is provided in alignment with our mission as a division of the American Chemical Society. | |
Explanation | The data from CAS Common Chemistry is provided under a CC-BY-NC 4.0 license, unless otherwise stated. | |
Record name | 3,5-Dimethylcyclohex-2-en-1-one | |
Source | ChemIDplus | |
URL | https://pubchem.ncbi.nlm.nih.gov/substance/?source=chemidplus&sourceid=0001123097 | |
Description | ChemIDplus is a free, web search system that provides access to the structure and nomenclature authority files used for the identification of chemical substances cited in National Library of Medicine (NLM) databases, including the TOXNET system. | |
Record name | 3,5-Dimethyl-2-cyclohexen-1-one | |
Source | DTP/NCI | |
URL | https://dtp.cancer.gov/dtpstandard/servlet/dwindex?searchtype=NSC&outputformat=html&searchlist=10113 | |
Description | The NCI Development Therapeutics Program (DTP) provides services and resources to the academic and private-sector research communities worldwide to facilitate the discovery and development of new cancer therapeutic agents. | |
Explanation | Unless otherwise indicated, all text within NCI products is free of copyright and may be reused without our permission. Credit the National Cancer Institute as the source. | |
Record name | 3,5-Dimethyl-2-cyclohexen-1-one | |
Source | DTP/NCI | |
URL | https://dtp.cancer.gov/dtpstandard/servlet/dwindex?searchtype=NSC&outputformat=html&searchlist=845 | |
Description | The NCI Development Therapeutics Program (DTP) provides services and resources to the academic and private-sector research communities worldwide to facilitate the discovery and development of new cancer therapeutic agents. | |
Explanation | Unless otherwise indicated, all text within NCI products is free of copyright and may be reused without our permission. Credit the National Cancer Institute as the source. | |
Record name | 3,5-Dimethylcyclohex-2-en-1-one | |
Source | EPA DSSTox | |
URL | https://comptox.epa.gov/dashboard/DTXSID20870848 | |
Description | DSSTox provides a high quality public chemistry resource for supporting improved predictive toxicology. | |
Record name | 3,5-dimethylcyclohex-2-en-1-one | |
Source | European Chemicals Agency (ECHA) | |
URL | https://echa.europa.eu/substance-information/-/substanceinfo/100.013.064 | |
Description | The European Chemicals Agency (ECHA) is an agency of the European Union which is the driving force among regulatory authorities in implementing the EU's groundbreaking chemicals legislation for the benefit of human health and the environment as well as for innovation and competitiveness. | |
Explanation | Use of the information, documents and data from the ECHA website is subject to the terms and conditions of this Legal Notice, and subject to other binding limitations provided for under applicable law, the information, documents and data made available on the ECHA website may be reproduced, distributed and/or used, totally or in part, for non-commercial purposes provided that ECHA is acknowledged as the source: "Source: European Chemicals Agency, http://echa.europa.eu/". Such acknowledgement must be included in each copy of the material. ECHA permits and encourages organisations and individuals to create links to the ECHA website under the following cumulative conditions: Links can only be made to webpages that provide a link to the Legal Notice page. | |
Q1: What are some of the key applications of 3,5-dimethyl-2-cyclohexen-1-one in organic synthesis?
A1: this compound serves as a valuable building block in organic synthesis. For instance, it can be used as a starting material for synthesizing alkyl-substituted enol lactone systems, which are known inhibitors of the serine protease elastase []. This enzyme plays a significant role in various physiological processes, making its inhibitors potential therapeutic targets. Additionally, this compound plays a crucial role in synthesizing syn-4,6-dimethyldodecanal, identified as the male sex pheromone and trail-following pheromone in two termite species, Zootermopsis nevadensis and Zootermopsis angusticollis []. This finding has implications for understanding chemical communication and behavior in these insects and potentially for developing pest control strategies.
Q2: How can this compound be utilized in the development of new materials with interesting optical properties?
A2: Research shows that incorporating this compound into specific molecular frameworks can lead to materials with highly nonlinear optical (NLO) properties []. For example, researchers have synthesized single crystals of 2-(5-methyl-3-(4-(pyrrolidin-1-yl)styryl)cyclohex-2-enylidene)malononitrile (MH2) utilizing a this compound scaffold. These crystals display significant macroscopic nonlinearity, comparable to the well-studied DAST, highlighting their potential for applications in optoelectronics and photonics.
Q3: Can microorganisms be used to modify the structure of this compound?
A3: Yes, studies have demonstrated the successful microbiological oxidation of isophorone (a close structural analogue of this compound) to 4-hydroxyisophorone []. This highlights the potential for utilizing microbial biotransformations to introduce specific functional groups into the this compound structure. Such modifications can be valuable for accessing new derivatives with altered reactivity and potential applications in various fields.
Q4: Are there any known analytical methods for characterizing and quantifying this compound and its derivatives?
A4: While the provided abstracts don't offer detailed information on specific analytical techniques, gas chromatography-mass spectrometry (GC-MS) is likely employed for the analysis of this compound and its derivatives, particularly in the context of pheromone research []. Additionally, techniques like nuclear magnetic resonance (NMR) spectroscopy and high-performance liquid chromatography (HPLC) are likely employed for structural characterization and quantification, especially when studying synthetic pathways and reaction optimization [, ].
Disclaimer and Information on In-Vitro Research Products
Please be aware that all articles and product information presented on BenchChem are intended solely for informational purposes. The products available for purchase on BenchChem are specifically designed for in-vitro studies, which are conducted outside of living organisms. In-vitro studies, derived from the Latin term "in glass," involve experiments performed in controlled laboratory settings using cells or tissues. It is important to note that these products are not categorized as medicines or drugs, and they have not received approval from the FDA for the prevention, treatment, or cure of any medical condition, ailment, or disease. We must emphasize that any form of bodily introduction of these products into humans or animals is strictly prohibited by law. It is essential to adhere to these guidelines to ensure compliance with legal and ethical standards in research and experimentation.