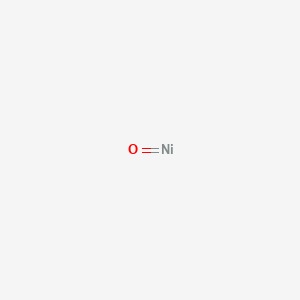
Nickel(II) oxide
- Click on QUICK INQUIRY to receive a quote from our team of experts.
- With the quality product at a COMPETITIVE price, you can focus more on your research.
Overview
Description
Nickel(II) oxide (NiO) is a chemical compound that is the principal oxide of nickel. It is classified as a basic metal oxide and is produced in several million kilograms annually, primarily as an intermediate in the production of nickel alloys .
Mechanism of Action
Target of Action
Nickel oxide (NiO) is a p-type metal oxide that plays a significant role in various optoelectronic devices . The primary targets of NiO are the electrochromic materials in these devices . The widely accepted electrochromic mechanism of NiO involves the reversible conversion between colorless Ni 2+ and colored Ni 3+ and Ni 4+ .
Mode of Action
NiO interacts with its targets by undergoing a reversible conversion between its colorless (Ni 2+) and colored (Ni 3+ and Ni 4+) states . This interaction results in changes to the optical properties of the device, such as its color. The performance of NiO can be improved by optimizing the preparation parameters, which can adjust the morphology, crystal structure, and chemical state of the NiO film .
Biochemical Pathways
NiO toxicity can induce reactive oxygen species accumulation and oxidative stress responses in plants . It also reduces the phytohormone concentrations, including auxin, cytokinin, and gibberellic acid, thereby retarding plant growth . NiO toxicity alters the expression of genes involved in carbon/nitrogen metabolism pathways .
Pharmacokinetics
The pharmacokinetics of NiO nanoparticles (NPs) have been studied in the context of liver cancer treatment . NiO NPs are used as photosensitizers due to their significant bioavailability . HepG2 cells were incubated with different concentrations of NiO NPs ranging from 0-80μg/ml at 37 °C for 24 hours .
Result of Action
The result of NiO’s action depends on its application. In optoelectronic devices, NiO’s action results in dynamically adjustable optical properties, which can reduce building energy consumption . In a biological context, NiO toxicity can lead to organ damage by triggering downstream signaling through various pathways .
Action Environment
The action, efficacy, and stability of NiO can be influenced by environmental factors. For instance, NiO’s electrochromic performance can be enhanced by using appropriate modification strategies, such as surface modification, nanomaterialization, material compounding, doping, and electrolyte design . In a biological context, NiO’s toxicity can be influenced by factors such as the concentration of NiO NPs and the duration of exposure .
Biochemical Analysis
Biochemical Properties
Nickel oxide has been shown to interact with various biomolecules. For instance, a study on the ground beetle Blaps polychresta revealed that nickel oxide nanoparticles induced significant changes in aspartate aminotransferase (AST) and alanine aminotransferase (ALT) activities . These enzymes play crucial roles in amino acid metabolism, indicating that nickel oxide can influence biochemical reactions involving these enzymes .
Cellular Effects
Nickel oxide has been observed to have various effects on cells. In the aforementioned study, nickel oxide nanoparticles were found to cause histological alterations in the midgut tissues of the ground beetle . This suggests that nickel oxide can influence cell function and potentially impact cellular metabolism .
Molecular Mechanism
The molecular mechanism of nickel oxide’s action is complex and multifaceted. It appears to exert its effects at the molecular level through interactions with various biomolecules, leading to changes in enzyme activity and potentially influencing gene expression . The exact nature of these interactions and their consequences are still being explored.
Temporal Effects in Laboratory Settings
In laboratory settings, the effects of nickel oxide have been observed to change over time. For instance, the study on the ground beetle showed that the effects of nickel oxide nanoparticles on AST and ALT activities varied over a 30-day period . This suggests that nickel oxide may have long-term effects on cellular function .
Dosage Effects in Animal Models
The effects of nickel oxide also appear to vary with dosage. In the ground beetle study, different doses of nickel oxide nanoparticles were tested, and the sublethal dose of 0.02 mg/g was selected for further testing . This indicates that the effects of nickel oxide can be dose-dependent .
Metabolic Pathways
Its interaction with AST and ALT suggests that it may influence amino acid metabolism
Transport and Distribution
It is known that nickel oxide nanoparticles can be detected in midgut tissues, suggesting that they can be transported to and accumulate in specific tissues .
Subcellular Localization
The subcellular localization of nickel oxide is another area of active research. The presence of nickel oxide nanoparticles in midgut tissues suggests that they may be localized within specific cellular compartments .
Preparation Methods
Nickel(II) oxide can be prepared through various methods:
Synthetic Routes and Reaction Conditions:
Industrial Production Methods:
Chemical Reactions Analysis
Nickel(II) oxide undergoes various types of reactions:
Oxidation and Reduction:
Substitution: This compound reacts with the oxides of sodium and potassium at high temperatures to form corresponding nickelates.
Common Reagents and Conditions:
Major Products:
Scientific Research Applications
Nickel(II) oxide has a wide range of applications in scientific research:
Comparison with Similar Compounds
Nickel(II) oxide can be compared with other similar compounds:
Nickel(III) Oxide (Ni2O3): A higher oxidation state of nickel oxide.
Nickel Hydroxide (Ni(OH)2): Used in nickel-cadmium and nickel-metal hydride batteries.
Nickel Carbonate (NiCO3): Another nickel compound that can be reduced to nickel oxide.
This compound’s diverse applications and unique properties make it a valuable compound in various scientific and industrial fields.
Properties
CAS No. |
1313-99-1 |
---|---|
Molecular Formula |
NiO |
Molecular Weight |
74.693 g/mol |
IUPAC Name |
nickel(2+);oxygen(2-) |
InChI |
InChI=1S/Ni.O/q+2;-2 |
InChI Key |
KVAWBJDCAFOHRG-UHFFFAOYSA-N |
impurities |
Typical impurities, present at levels of 1% or less, include cobalt, copper, iron, and sulfur. |
SMILES |
O=[Ni] |
Canonical SMILES |
[O-2].[Ni+2] |
Color/Form |
Green powder Greenish-black cubic crystals Yellow when hot |
density |
6.67 (NTP, 1992) 6.72 6.7 g/cm³ |
melting_point |
3603 °F (NTP, 1992) 1955 °C |
Key on ui other cas no. |
1313-99-1 34492-97-2 11099-02-8 |
physical_description |
Nickel oxide appears as odorless green-black cubic crystals (yellow when hot) or green powder. (NTP, 1992) DryPowder DryPowder; OtherSolid; PelletsLargeCrystals; WetSolid GREEN-TO-BLACK CRYSTALLINE POWDER. |
Pictograms |
Irritant; Health Hazard; Environmental Hazard |
solubility |
less than 1 mg/mL at 68° F (NTP, 1992) 0.11 MG/100 ML @ 20 °C SOL IN ACIDS; AMMONIUM HYDROXIDE Insol in caustic solutions SOL IN POTASSIUM CYANIDE Insoluble in water; soluble in acids Solubility in water, mg/l at 20 °C: 1.1 (practically insoluble) |
Synonyms |
nickel monoxide nickel oxide nickel(II)oxide |
vapor_pressure |
0 mm Hg at 68 °F (NTP, 1992) |
Origin of Product |
United States |
Disclaimer and Information on In-Vitro Research Products
Please be aware that all articles and product information presented on BenchChem are intended solely for informational purposes. The products available for purchase on BenchChem are specifically designed for in-vitro studies, which are conducted outside of living organisms. In-vitro studies, derived from the Latin term "in glass," involve experiments performed in controlled laboratory settings using cells or tissues. It is important to note that these products are not categorized as medicines or drugs, and they have not received approval from the FDA for the prevention, treatment, or cure of any medical condition, ailment, or disease. We must emphasize that any form of bodily introduction of these products into humans or animals is strictly prohibited by law. It is essential to adhere to these guidelines to ensure compliance with legal and ethical standards in research and experimentation.