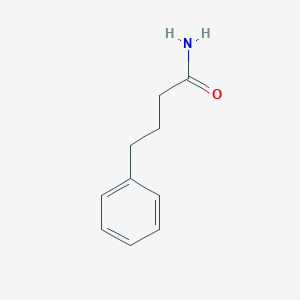
4-Phenylbutanamide
Overview
Description
4-Phenylbutanamide is an organic compound with the molecular formula C10H13NO. It is a derivative of butanamide, where a phenyl group is attached to the fourth carbon atom of the butanamide chain. This compound is known for its applications in various fields, including pharmaceuticals and organic synthesis.
Biochemical Analysis
Biochemical Properties
4-Phenylbutanamide has been found to interact with certain enzymes and proteins. For instance, it has been identified as a Histone Deacetylase (HDAC) inhibitor . HDACs are enzymes that remove acetyl groups from an ε-N-acetyl lysine amino acid on a histone, allowing the histones to wrap the DNA more tightly. By inhibiting HDACs, this compound can affect gene expression and protein function .
Cellular Effects
This compound has shown to have significant effects on cellular processes. It has been found to exhibit neuroprotective effects, protecting against neuronal cell death by primarily acting as a chemical chaperone . It also exhibits inhibitory activity against histone deacetylases (HDACs), which can influence cell function, including impact on cell signaling pathways, gene expression, and cellular metabolism .
Molecular Mechanism
The molecular mechanism of this compound involves its interaction with biomolecules at the molecular level. As an HDAC inhibitor, it can bind to these enzymes and inhibit their activity, leading to changes in gene expression . It also exerts its effects by ameliorating unfolded proteins and suppressing their aggregation, which results in protective effects against endoplasmic reticulum stress-induced neuronal cell death .
Temporal Effects in Laboratory Settings
It is known that the compound exhibits long-term effects on cellular function, particularly in the context of neurodegenerative diseases .
Dosage Effects in Animal Models
While specific dosage effects of this compound in animal models are not fully documented, it is known that the compound has been used in studies involving animal models of neurodegenerative diseases
Metabolic Pathways
As an HDAC inhibitor, it is likely to be involved in pathways related to gene expression and protein function .
Subcellular Localization
Given its role as an HDAC inhibitor, it is likely to be found in the nucleus where it can interact with histones and influence gene expression .
Preparation Methods
Synthetic Routes and Reaction Conditions: 4-Phenylbutanamide can be synthesized through several methods. One common method involves the reaction of 4-phenylbutyric acid with ammonia or an amine under dehydrating conditions. The reaction typically requires a catalyst such as dicyclohexylcarbodiimide (DCC) and is carried out in an organic solvent like dichloromethane.
Industrial Production Methods: In industrial settings, this compound is often produced through catalytic hydrogenation of 4-phenylbutyronitrile. This process involves the use of a metal catalyst such as palladium on carbon (Pd/C) under high pressure and temperature conditions.
Chemical Reactions Analysis
Types of Reactions: 4-Phenylbutanamide undergoes various chemical reactions, including:
Oxidation: It can be oxidized to form 4-phenylbutanoic acid using oxidizing agents like potassium permanganate (KMnO4).
Reduction: Reduction of this compound can yield 4-phenylbutylamine using reducing agents such as lithium aluminum hydride (LiAlH4).
Substitution: The amide group can participate in nucleophilic substitution reactions, where the amide nitrogen is replaced by other nucleophiles.
Common Reagents and Conditions:
Oxidation: Potassium permanganate (KMnO4) in an aqueous medium.
Reduction: Lithium aluminum hydride (LiAlH4) in anhydrous ether.
Substitution: Various nucleophiles under acidic or basic conditions.
Major Products Formed:
Oxidation: 4-Phenylbutanoic acid.
Reduction: 4-Phenylbutylamine.
Substitution: Depending on the nucleophile, various substituted amides can be formed.
Scientific Research Applications
4-Phenylbutanamide has several applications in scientific research:
Chemistry: It is used as an intermediate in the synthesis of more complex organic molecules.
Biology: It serves as a building block for the synthesis of biologically active compounds.
Medicine: Derivatives of this compound have been studied for their potential therapeutic effects, including anti-cancer and anti-inflammatory properties.
Industry: It is used in the production of specialty chemicals and pharmaceuticals.
Mechanism of Action
The mechanism of action of 4-Phenylbutanamide and its derivatives often involves interaction with specific molecular targets. For example, some derivatives act as inhibitors of histone deacetylase 6 (HDAC6), an enzyme involved in the regulation of gene expression. By inhibiting HDAC6, these compounds can induce cell cycle arrest and apoptosis in cancer cells.
Comparison with Similar Compounds
4-Phenylbutyric acid: A precursor in the synthesis of 4-Phenylbutanamide.
4-Phenylbutylamine: A reduction product of this compound.
N-Phenylbutyramide: Another amide derivative with similar structural features.
Uniqueness: this compound is unique due to its specific structural configuration, which allows it to participate in a variety of chemical reactions and exhibit diverse biological activities. Its ability to act as an HDAC6 inhibitor distinguishes it from other similar compounds, making it a valuable molecule in medicinal chemistry research.
Properties
IUPAC Name |
4-phenylbutanamide | |
---|---|---|
Source | PubChem | |
URL | https://pubchem.ncbi.nlm.nih.gov | |
Description | Data deposited in or computed by PubChem | |
InChI |
InChI=1S/C10H13NO/c11-10(12)8-4-7-9-5-2-1-3-6-9/h1-3,5-6H,4,7-8H2,(H2,11,12) | |
Source | PubChem | |
URL | https://pubchem.ncbi.nlm.nih.gov | |
Description | Data deposited in or computed by PubChem | |
InChI Key |
LEPWUMPXISBPIB-UHFFFAOYSA-N | |
Source | PubChem | |
URL | https://pubchem.ncbi.nlm.nih.gov | |
Description | Data deposited in or computed by PubChem | |
Canonical SMILES |
C1=CC=C(C=C1)CCCC(=O)N | |
Source | PubChem | |
URL | https://pubchem.ncbi.nlm.nih.gov | |
Description | Data deposited in or computed by PubChem | |
Molecular Formula |
C10H13NO | |
Source | PubChem | |
URL | https://pubchem.ncbi.nlm.nih.gov | |
Description | Data deposited in or computed by PubChem | |
DSSTOX Substance ID |
DTXSID50294735 | |
Record name | 4-phenylbutanamide | |
Source | EPA DSSTox | |
URL | https://comptox.epa.gov/dashboard/DTXSID50294735 | |
Description | DSSTox provides a high quality public chemistry resource for supporting improved predictive toxicology. | |
Molecular Weight |
163.22 g/mol | |
Source | PubChem | |
URL | https://pubchem.ncbi.nlm.nih.gov | |
Description | Data deposited in or computed by PubChem | |
CAS No. |
1199-98-0 | |
Record name | 1199-98-0 | |
Source | DTP/NCI | |
URL | https://dtp.cancer.gov/dtpstandard/servlet/dwindex?searchtype=NSC&outputformat=html&searchlist=97770 | |
Description | The NCI Development Therapeutics Program (DTP) provides services and resources to the academic and private-sector research communities worldwide to facilitate the discovery and development of new cancer therapeutic agents. | |
Explanation | Unless otherwise indicated, all text within NCI products is free of copyright and may be reused without our permission. Credit the National Cancer Institute as the source. | |
Record name | 4-phenylbutanamide | |
Source | EPA DSSTox | |
URL | https://comptox.epa.gov/dashboard/DTXSID50294735 | |
Description | DSSTox provides a high quality public chemistry resource for supporting improved predictive toxicology. | |
Record name | 4-PHENYLBUTYRAMIDE | |
Source | European Chemicals Agency (ECHA) | |
URL | https://echa.europa.eu/information-on-chemicals | |
Description | The European Chemicals Agency (ECHA) is an agency of the European Union which is the driving force among regulatory authorities in implementing the EU's groundbreaking chemicals legislation for the benefit of human health and the environment as well as for innovation and competitiveness. | |
Explanation | Use of the information, documents and data from the ECHA website is subject to the terms and conditions of this Legal Notice, and subject to other binding limitations provided for under applicable law, the information, documents and data made available on the ECHA website may be reproduced, distributed and/or used, totally or in part, for non-commercial purposes provided that ECHA is acknowledged as the source: "Source: European Chemicals Agency, http://echa.europa.eu/". Such acknowledgement must be included in each copy of the material. ECHA permits and encourages organisations and individuals to create links to the ECHA website under the following cumulative conditions: Links can only be made to webpages that provide a link to the Legal Notice page. | |
Retrosynthesis Analysis
AI-Powered Synthesis Planning: Our tool employs the Template_relevance Pistachio, Template_relevance Bkms_metabolic, Template_relevance Pistachio_ringbreaker, Template_relevance Reaxys, Template_relevance Reaxys_biocatalysis model, leveraging a vast database of chemical reactions to predict feasible synthetic routes.
One-Step Synthesis Focus: Specifically designed for one-step synthesis, it provides concise and direct routes for your target compounds, streamlining the synthesis process.
Accurate Predictions: Utilizing the extensive PISTACHIO, BKMS_METABOLIC, PISTACHIO_RINGBREAKER, REAXYS, REAXYS_BIOCATALYSIS database, our tool offers high-accuracy predictions, reflecting the latest in chemical research and data.
Strategy Settings
Precursor scoring | Relevance Heuristic |
---|---|
Min. plausibility | 0.01 |
Model | Template_relevance |
Template Set | Pistachio/Bkms_metabolic/Pistachio_ringbreaker/Reaxys/Reaxys_biocatalysis |
Top-N result to add to graph | 6 |
Feasible Synthetic Routes
A: Research suggests that certain 4-Phenylbutanamide derivatives, specifically those incorporating a benzothiazinone moiety, demonstrate potent inhibitory activity against both the adenosine A2A receptor (A2AAR) and monoamine oxidase B (MAO-B). [] This dual inhibition is particularly relevant in the context of neurodegenerative diseases like Parkinson's disease. Blocking A2AARs can help modulate dopamine release, while inhibiting MAO-B can prevent dopamine breakdown, both potentially contributing to improved motor function. []
A: this compound (IIb) is structurally similar to the anticonvulsant molecule 4-hydroxy-4-phenylhexanamide (I). []
A: Studies exploring the SAR of this compound derivatives, specifically in the context of A2AAR and MAO-B inhibition, highlight the importance of substituents. For instance, large substituents at the 2-position of benzothiazinones, such as methoxycinnamoylamino or phenylbutyrylamino residues, contribute to high affinity and selectivity for A2AARs. [] Conversely, smaller substituents at the same position, like a 3-chlorophenoxy group, appear to favor MAO-B inhibition. [] This suggests that subtle structural modifications can significantly alter the target selectivity and overall pharmacological activity of this compound derivatives.
A: While specific information on this compound's inherent stability is limited within the provided literature, researchers have successfully synthesized and evaluated various derivatives. [, , , , ] This suggests that appropriate synthetic strategies and potentially specific formulation approaches can be employed to handle and utilize this compound.
A: While not explicitly mentioned for this compound itself, various analytical techniques are frequently used to characterize and study similar compounds. These include spectroscopic methods like FTIR, 1H NMR, 13C NMR, and 119Sn NMR, which provide valuable information about the structure and bonding within the molecule. [] Additionally, techniques like GC-MS are valuable for identifying and quantifying aroma components in complex mixtures, such as those found in pineapple fruits. []
A: Yes, computational chemistry has played a significant role in understanding the interactions of this compound derivatives with their targets. Molecular docking studies, for example, have been employed to investigate the binding modes of these compounds within the active site of HDAC6, providing insights into their potential as inhibitors. [] Additionally, molecular dynamics simulations, often coupled with methods like MMGBSA, offer a dynamic view of these interactions, shedding light on the stability of the formed complexes and the key residues involved in binding. []
ANone: The provided literature excerpts do not contain specific data on the toxicity profile of this compound. It's crucial to acknowledge that any potential therapeutic application would necessitate a thorough toxicological evaluation. This would involve assessing acute and chronic toxicity in preclinical models, identifying potential target organs, and determining safe dosing ranges.
A: While a detailed historical account is absent from the provided literature, research on this compound and its derivatives appears to be an evolving field. Initial interest stemmed from its structural similarity to known anticonvulsant compounds. [] Subsequent investigations have explored its potential in diverse therapeutic areas, including anticancer [] and neuroprotective applications, [] highlighting its versatility as a scaffold for drug discovery.
Disclaimer and Information on In-Vitro Research Products
Please be aware that all articles and product information presented on BenchChem are intended solely for informational purposes. The products available for purchase on BenchChem are specifically designed for in-vitro studies, which are conducted outside of living organisms. In-vitro studies, derived from the Latin term "in glass," involve experiments performed in controlled laboratory settings using cells or tissues. It is important to note that these products are not categorized as medicines or drugs, and they have not received approval from the FDA for the prevention, treatment, or cure of any medical condition, ailment, or disease. We must emphasize that any form of bodily introduction of these products into humans or animals is strictly prohibited by law. It is essential to adhere to these guidelines to ensure compliance with legal and ethical standards in research and experimentation.