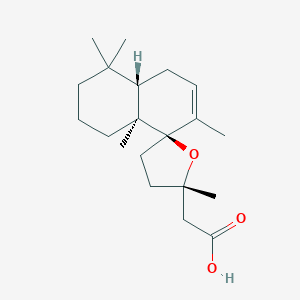
Grindelic acid
Overview
Description
Grindelic acid is a diterpenoid compound predominantly found in the resinous exudates of plants belonging to the genus Grindelia, such as Grindelia robusta and Grindelia squarrosa . This compound has garnered attention due to its potential pharmaceutical applications and its role in plant defense mechanisms .
Mechanism of Action
Target of Action
Grindelic acid primarily targets the respiratory epithelium and human macrophages . It modulates the pro-inflammatory functions of these cells, which play a crucial role in the body’s response to bacterial infection .
Mode of Action
This compound interacts with its targets by modulating the expression of surface receptors such as TLR-4 and the concentration of the NF-κB transcription factor . This modulation leads to a decrease in the production of a wide spectrum of cytokines, including TNF-α, IL-1β, IL-6, and IL-8 .
Biochemical Pathways
The primary biochemical pathway affected by this compound is the NF-κB signaling pathway . By decreasing the concentration of the NF-κB transcription factor, this compound inhibits the synthesis of pro-inflammatory cytokines . This modulation of the NF-κB pathway results in a decrease in inflammation and an improvement in symptoms related to cold and other respiratory diseases .
Pharmacokinetics
It is known that this compound is used in cell suspension cultures, suggesting that it can be absorbed and distributed in the body
Result of Action
The primary result of this compound’s action is a significant modulation of pro-inflammatory functions in the respiratory epithelium . This includes a decrease in the expression of TLR-4, a reduction in the concentration of the NF-κB transcription factor, and an inhibition of cytokine synthesis . These molecular and cellular effects contribute to the compound’s potential therapeutic benefits in treating respiratory diseases .
Action Environment
The action of this compound can be influenced by environmental factors. For example, studies have shown that the accumulation of this compound in Grindelia pulchella cell suspension cultures can be affected by the presence of abiotic elicitors such as CuSO4 . This suggests that the compound’s action, efficacy, and stability may vary depending on the environmental conditions.
Biochemical Analysis
Biochemical Properties
Grindelic acid is involved in various biochemical reactions. This enzyme converts GGPP into labda-7,13E-dienyl diphosphate, which is suggested to be the first committed reaction in the biosynthesis of this compound .
Cellular Effects
This compound has been shown to modulate pro-inflammatory functions of respiratory epithelium and human macrophages . Specifically, it has been observed to decrease TLR-4 expression and p65 NF-κB concentration, leading to the inhibition of cytokine synthesis (IL-8, TNF-α, IL-1β, and IL-6) in both cellular models .
Molecular Mechanism
At the molecular level, this compound exerts its effects through selective modulation of respiratory epithelium functions. It has been observed to affect TLR-4 expression, cytokine production, and p65 NF-κB concentration . Interestingly, this compound does not affect adhesive molecules expression and pro-inflammatory functions of macrophages .
Temporal Effects in Laboratory Settings
In laboratory settings, the effects of this compound have been observed to change over time. For instance, in cell suspension cultures of Grindelia pulchella elicited with CuSO4, this compound accumulation was initially inhibited but increased in the stationary phase .
Metabolic Pathways
This compound is involved in the diterpenoid biosynthesis pathway. The enzyme GrTPS2, a class II diTPS, plays a crucial role in this pathway by converting GGPP into labda-7,13E-dienyl diphosphate .
Preparation Methods
Synthetic Routes and Reaction Conditions: Grindelic acid can be synthesized through various methods, including cell suspension cultures of Grindelia pulchella . The process involves the use of media supplemented with specific growth regulators like indolbutiric acid and bencilamine purine. The production is often enhanced by the addition of abiotic elicitors such as copper sulfate .
Industrial Production Methods: Industrial production of this compound typically involves the extraction from plant material. The process includes acidifying the liquid media to a specific pH, followed by liquid-liquid extraction using solvents like diethyl ether. The cells are then extracted by reflux in methanol, and the methanolic extract is further processed to isolate this compound .
Chemical Reactions Analysis
Types of Reactions: Grindelic acid undergoes various chemical reactions, including oxidation, reduction, and substitution .
Common Reagents and Conditions:
Oxidation: Common oxidizing agents like sulfur trioxide-pyridine complex are used.
Reduction: Reducing agents such as diisobutylaluminum hydride (DIBAL-H) are employed.
Substitution: Reagents like ethyl 2-(triphenyl-phosphoranylidene)propanoate are used in substitution reactions.
Major Products: The reactions yield various derivatives of this compound, including synthetic antitumor derivatives .
Scientific Research Applications
Grindelic acid has a wide range of scientific research applications:
Comparison with Similar Compounds
Grindelic acid is unique among diterpenoids due to its specific structure and biological activities. Similar compounds include:
Manoyl oxide derivatives: These compounds share structural similarities and are also found in Grindelia species.
Other this compound derivatives: Such as 19-hydroxythis compound and methyl grindeloate, which have been isolated from various Grindelia species.
This compound stands out due to its specific anti-inflammatory and antitumor properties, making it a compound of significant interest in both scientific research and industrial applications.
Properties
IUPAC Name |
2-[(2'S,4aS,8R,8aS)-2',4,4,7,8a-pentamethylspiro[2,3,4a,5-tetrahydro-1H-naphthalene-8,5'-oxolane]-2'-yl]acetic acid | |
---|---|---|
Source | PubChem | |
URL | https://pubchem.ncbi.nlm.nih.gov | |
Description | Data deposited in or computed by PubChem | |
InChI |
InChI=1S/C20H32O3/c1-14-7-8-15-17(2,3)9-6-10-19(15,5)20(14)12-11-18(4,23-20)13-16(21)22/h7,15H,6,8-13H2,1-5H3,(H,21,22)/t15-,18-,19-,20+/m0/s1 | |
Source | PubChem | |
URL | https://pubchem.ncbi.nlm.nih.gov | |
Description | Data deposited in or computed by PubChem | |
InChI Key |
XLWWERNKTLITEF-MVJPYGJCSA-N | |
Source | PubChem | |
URL | https://pubchem.ncbi.nlm.nih.gov | |
Description | Data deposited in or computed by PubChem | |
Canonical SMILES |
CC1=CCC2C(CCCC2(C13CCC(O3)(C)CC(=O)O)C)(C)C | |
Source | PubChem | |
URL | https://pubchem.ncbi.nlm.nih.gov | |
Description | Data deposited in or computed by PubChem | |
Isomeric SMILES |
CC1=CC[C@@H]2[C@@]([C@@]13CC[C@@](O3)(C)CC(=O)O)(CCCC2(C)C)C | |
Source | PubChem | |
URL | https://pubchem.ncbi.nlm.nih.gov | |
Description | Data deposited in or computed by PubChem | |
Molecular Formula |
C20H32O3 | |
Source | PubChem | |
URL | https://pubchem.ncbi.nlm.nih.gov | |
Description | Data deposited in or computed by PubChem | |
Molecular Weight |
320.5 g/mol | |
Source | PubChem | |
URL | https://pubchem.ncbi.nlm.nih.gov | |
Description | Data deposited in or computed by PubChem | |
CAS No. |
1438-57-9 | |
Record name | Grindelic acid | |
Source | ChemIDplus | |
URL | https://pubchem.ncbi.nlm.nih.gov/substance/?source=chemidplus&sourceid=0001438579 | |
Description | ChemIDplus is a free, web search system that provides access to the structure and nomenclature authority files used for the identification of chemical substances cited in National Library of Medicine (NLM) databases, including the TOXNET system. | |
Record name | GRINDELIC ACID | |
Source | FDA Global Substance Registration System (GSRS) | |
URL | https://gsrs.ncats.nih.gov/ginas/app/beta/substances/4QX9FCD1IN | |
Description | The FDA Global Substance Registration System (GSRS) enables the efficient and accurate exchange of information on what substances are in regulated products. Instead of relying on names, which vary across regulatory domains, countries, and regions, the GSRS knowledge base makes it possible for substances to be defined by standardized, scientific descriptions. | |
Explanation | Unless otherwise noted, the contents of the FDA website (www.fda.gov), both text and graphics, are not copyrighted. They are in the public domain and may be republished, reprinted and otherwise used freely by anyone without the need to obtain permission from FDA. Credit to the U.S. Food and Drug Administration as the source is appreciated but not required. | |
Retrosynthesis Analysis
AI-Powered Synthesis Planning: Our tool employs the Template_relevance Pistachio, Template_relevance Bkms_metabolic, Template_relevance Pistachio_ringbreaker, Template_relevance Reaxys, Template_relevance Reaxys_biocatalysis model, leveraging a vast database of chemical reactions to predict feasible synthetic routes.
One-Step Synthesis Focus: Specifically designed for one-step synthesis, it provides concise and direct routes for your target compounds, streamlining the synthesis process.
Accurate Predictions: Utilizing the extensive PISTACHIO, BKMS_METABOLIC, PISTACHIO_RINGBREAKER, REAXYS, REAXYS_BIOCATALYSIS database, our tool offers high-accuracy predictions, reflecting the latest in chemical research and data.
Strategy Settings
Precursor scoring | Relevance Heuristic |
---|---|
Min. plausibility | 0.01 |
Model | Template_relevance |
Template Set | Pistachio/Bkms_metabolic/Pistachio_ringbreaker/Reaxys/Reaxys_biocatalysis |
Top-N result to add to graph | 6 |
Feasible Synthetic Routes
ANone: Grindelic acid, a diterpene acid found in Grindelia squarrosa, has been shown to modulate the pro-inflammatory functions of respiratory epithelial cells and macrophages. While its exact mechanism of action is not fully understood, research suggests that this compound may decrease the expression of TLR-4 receptors and reduce the concentration of the p65 NF-κB transcription factor in LPS-stimulated nasal and bronchial epithelial cells []. This, in turn, may lead to the inhibition of pro-inflammatory cytokine synthesis, including IL-8, TNF-α, IL-1β, and IL-6 [].
ANone: Yes, studies show that this compound can modulate the pro-inflammatory functions of macrophages, though less potently than the total Grindelia squarrosa extract []. Further research is needed to fully elucidate the specific mechanisms involved.
ANone: this compound does not appear to have a direct antimicrobial effect. Studies examining its activity against a range of bacterial strains found no significant inhibition [].
ANone: this compound has the molecular formula C20H32O2 and a molecular weight of 304.47 g/mol.
ANone: The structure of this compound has been extensively studied using various spectroscopic techniques, including Nuclear Magnetic Resonance (NMR) and Mass Spectrometry (MS) [, , , ]. These analyses have provided detailed information about the compound's structure, including the position of functional groups and stereochemistry.
ANone: The research provided focuses primarily on the biological activity and chemical synthesis of this compound. While its stability and compatibility with various materials are crucial for potential applications, these aspects have not been explicitly explored in the provided literature.
ANone: Based on the provided research, there is no evidence to suggest that this compound possesses any inherent catalytic properties.
ANone: Yes, molecular docking studies have been conducted to investigate the potential interaction of this compound with histone deacetylase (HDAC) enzymes []. These simulations have shown that this compound exhibits a binding mode similar to known HDAC inhibitors and displays good binding affinity []. This suggests a potential mechanism for its observed cytotoxic activity.
ANone: Studies have demonstrated that modifying the this compound structure can significantly alter its biological activity. For example, introducing a hydroxyl group at the C-17 or C-6 position through bromination and subsequent reactions leads to derivatives with altered properties []. Additionally, research has shown that the presence of specific functional groups, like the carboxyl group at C-7, is crucial for its biological activity []. Further SAR studies are needed to fully explore the relationship between structural modifications and their impact on this compound's activity, potency, and selectivity.
Disclaimer and Information on In-Vitro Research Products
Please be aware that all articles and product information presented on BenchChem are intended solely for informational purposes. The products available for purchase on BenchChem are specifically designed for in-vitro studies, which are conducted outside of living organisms. In-vitro studies, derived from the Latin term "in glass," involve experiments performed in controlled laboratory settings using cells or tissues. It is important to note that these products are not categorized as medicines or drugs, and they have not received approval from the FDA for the prevention, treatment, or cure of any medical condition, ailment, or disease. We must emphasize that any form of bodily introduction of these products into humans or animals is strictly prohibited by law. It is essential to adhere to these guidelines to ensure compliance with legal and ethical standards in research and experimentation.