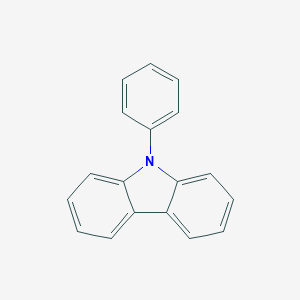
9-Phenylcarbazole
Overview
Description
9-Phenylcarbazole is an organic compound that belongs to the carbazole family. It consists of a carbazole core with a phenyl group attached at the ninth position. This compound is known for its unique photophysical properties, making it a valuable material in various scientific and industrial applications, particularly in the fields of optoelectronics and organic light-emitting diodes (OLEDs).
Mechanism of Action
Target of Action
9-Phenylcarbazole is a chemical compound with the formula C18H13N . It is primarily used in the field of optoelectronics . .
Mode of Action
The mode of action of this compound is largely based on its electrochemical properties . The oxidation potential of this compound is sensitive to any substituent at the 3- or 6-position on the carbazole . This suggests that the compound interacts with its targets through electron transfer processes .
Biochemical Pathways
It is known that the compound undergoes oxidation and reduction reactions . These reactions could potentially influence various biochemical pathways, particularly those involving electron transfer.
Result of Action
The result of this compound’s action is primarily observed in its applications in optoelectronics . For instance, it has been used in the synthesis of novel dendrimers, which exhibit high thermal stability, good amorphous properties, and deep blue emissions
Action Environment
The action of this compound can be influenced by various environmental factors. For example, the compound’s electrochemical properties, and thus its mode of action, can be affected by the presence of other chemical substances . .
Biochemical Analysis
Biochemical Properties
The biochemical properties of 9-Phenylcarbazole are largely influenced by its molecular structure
Molecular Mechanism
It is known that enhancing spin-orbital coupling by heteroatom and heavy-atom effects plays a critical role in its phosphorescence
Temporal Effects in Laboratory Settings
In laboratory settings, this compound has demonstrated high thermal stability
Preparation Methods
Synthetic Routes and Reaction Conditions: The synthesis of 9-Phenylcarbazole typically involves the reaction of carbazole with bromobenzene in the presence of a palladium catalyst. This process is known as the Suzuki coupling reaction. The reaction conditions generally include the use of a base such as potassium carbonate and a solvent like toluene. The reaction is carried out under an inert atmosphere, typically nitrogen or argon, at elevated temperatures.
Industrial Production Methods: On an industrial scale, the production of this compound follows similar synthetic routes but with optimized conditions to enhance yield and purity. The use of continuous flow reactors and advanced purification techniques such as recrystallization and chromatography are common to ensure high-quality production.
Types of Reactions:
Oxidation: this compound can undergo oxidation reactions, leading to the formation of various oxidized products. Common oxidizing agents include potassium permanganate and silver oxide.
Reduction: Reduction reactions of this compound are less common but can be achieved using reducing agents like lithium aluminum hydride.
Substitution: The compound can participate in electrophilic substitution reactions, particularly at the phenyl ring. Halogenation and nitration are typical examples.
Common Reagents and Conditions:
Oxidation: Potassium permanganate in acetone, silver oxide.
Reduction: Lithium aluminum hydride in anhydrous ether.
Substitution: Halogenation using bromine or chlorine in the presence of a catalyst.
Major Products:
Oxidation: Formation of 9,9’-bicarbazyl and other oligomers.
Reduction: Formation of reduced carbazole derivatives.
Substitution: Halogenated or nitrated this compound derivatives.
Scientific Research Applications
9-Phenylcarbazole has a wide range of applications in scientific research:
Optoelectronics: Used in the development of OLEDs due to its excellent photophysical properties.
Organic Photovoltaics: Employed as a material in organic solar cells.
Electrochemical Sensors: Utilized in the fabrication of sensors for detecting various analytes.
Biological Research: Investigated for its potential use in bioimaging and as a fluorescent probe.
Material Science: Used in the synthesis of polymers and other advanced materials with unique electronic properties.
Comparison with Similar Compounds
Carbazole: The parent compound of 9-Phenylcarbazole, known for its use in photoconductors and organic semiconductors.
9-Ethylcarbazole: Similar in structure but with an ethyl group instead of a phenyl group, used in similar applications.
9-Methylcarbazole: Another derivative with a methyl group, used in the synthesis of polymers and as a photoconductor.
Uniqueness of this compound: this compound stands out due to its enhanced photophysical properties, particularly its ability to undergo efficient intersystem crossing and form stable triplet excitons. This makes it a superior material for applications in OLEDs and other optoelectronic devices compared to its analogs.
Properties
IUPAC Name |
9-phenylcarbazole | |
---|---|---|
Source | PubChem | |
URL | https://pubchem.ncbi.nlm.nih.gov | |
Description | Data deposited in or computed by PubChem | |
InChI |
InChI=1S/C18H13N/c1-2-8-14(9-3-1)19-17-12-6-4-10-15(17)16-11-5-7-13-18(16)19/h1-13H | |
Source | PubChem | |
URL | https://pubchem.ncbi.nlm.nih.gov | |
Description | Data deposited in or computed by PubChem | |
InChI Key |
VIJYEGDOKCKUOL-UHFFFAOYSA-N | |
Source | PubChem | |
URL | https://pubchem.ncbi.nlm.nih.gov | |
Description | Data deposited in or computed by PubChem | |
Canonical SMILES |
C1=CC=C(C=C1)N2C3=CC=CC=C3C4=CC=CC=C42 | |
Source | PubChem | |
URL | https://pubchem.ncbi.nlm.nih.gov | |
Description | Data deposited in or computed by PubChem | |
Molecular Formula |
C18H13N | |
Source | PubChem | |
URL | https://pubchem.ncbi.nlm.nih.gov | |
Description | Data deposited in or computed by PubChem | |
DSSTOX Substance ID |
DTXSID50150928 | |
Record name | N-Phenylcarbazole | |
Source | EPA DSSTox | |
URL | https://comptox.epa.gov/dashboard/DTXSID50150928 | |
Description | DSSTox provides a high quality public chemistry resource for supporting improved predictive toxicology. | |
Molecular Weight |
243.3 g/mol | |
Source | PubChem | |
URL | https://pubchem.ncbi.nlm.nih.gov | |
Description | Data deposited in or computed by PubChem | |
Physical Description |
Solid; [Sigma-Aldrich MSDS] | |
Record name | N-Phenylcarbazole | |
Source | Haz-Map, Information on Hazardous Chemicals and Occupational Diseases | |
URL | https://haz-map.com/Agents/16070 | |
Description | Haz-Map® is an occupational health database designed for health and safety professionals and for consumers seeking information about the adverse effects of workplace exposures to chemical and biological agents. | |
Explanation | Copyright (c) 2022 Haz-Map(R). All rights reserved. Unless otherwise indicated, all materials from Haz-Map are copyrighted by Haz-Map(R). No part of these materials, either text or image may be used for any purpose other than for personal use. Therefore, reproduction, modification, storage in a retrieval system or retransmission, in any form or by any means, electronic, mechanical or otherwise, for reasons other than personal use, is strictly prohibited without prior written permission. | |
CAS No. |
1150-62-5 | |
Record name | 9-Phenylcarbazole | |
Source | CAS Common Chemistry | |
URL | https://commonchemistry.cas.org/detail?cas_rn=1150-62-5 | |
Description | CAS Common Chemistry is an open community resource for accessing chemical information. Nearly 500,000 chemical substances from CAS REGISTRY cover areas of community interest, including common and frequently regulated chemicals, and those relevant to high school and undergraduate chemistry classes. This chemical information, curated by our expert scientists, is provided in alignment with our mission as a division of the American Chemical Society. | |
Explanation | The data from CAS Common Chemistry is provided under a CC-BY-NC 4.0 license, unless otherwise stated. | |
Record name | N-Phenylcarbazole | |
Source | ChemIDplus | |
URL | https://pubchem.ncbi.nlm.nih.gov/substance/?source=chemidplus&sourceid=0001150625 | |
Description | ChemIDplus is a free, web search system that provides access to the structure and nomenclature authority files used for the identification of chemical substances cited in National Library of Medicine (NLM) databases, including the TOXNET system. | |
Record name | 9-Phenylcarbazole | |
Source | DTP/NCI | |
URL | https://dtp.cancer.gov/dtpstandard/servlet/dwindex?searchtype=NSC&outputformat=html&searchlist=10416 | |
Description | The NCI Development Therapeutics Program (DTP) provides services and resources to the academic and private-sector research communities worldwide to facilitate the discovery and development of new cancer therapeutic agents. | |
Explanation | Unless otherwise indicated, all text within NCI products is free of copyright and may be reused without our permission. Credit the National Cancer Institute as the source. | |
Record name | N-Phenylcarbazole | |
Source | EPA DSSTox | |
URL | https://comptox.epa.gov/dashboard/DTXSID50150928 | |
Description | DSSTox provides a high quality public chemistry resource for supporting improved predictive toxicology. | |
Record name | N-phenylcarbazole | |
Source | European Chemicals Agency (ECHA) | |
URL | https://echa.europa.eu/substance-information/-/substanceinfo/100.013.240 | |
Description | The European Chemicals Agency (ECHA) is an agency of the European Union which is the driving force among regulatory authorities in implementing the EU's groundbreaking chemicals legislation for the benefit of human health and the environment as well as for innovation and competitiveness. | |
Explanation | Use of the information, documents and data from the ECHA website is subject to the terms and conditions of this Legal Notice, and subject to other binding limitations provided for under applicable law, the information, documents and data made available on the ECHA website may be reproduced, distributed and/or used, totally or in part, for non-commercial purposes provided that ECHA is acknowledged as the source: "Source: European Chemicals Agency, http://echa.europa.eu/". Such acknowledgement must be included in each copy of the material. ECHA permits and encourages organisations and individuals to create links to the ECHA website under the following cumulative conditions: Links can only be made to webpages that provide a link to the Legal Notice page. | |
Synthesis routes and methods I
Procedure details
Synthesis routes and methods II
Procedure details
Synthesis routes and methods III
Procedure details
Synthesis routes and methods IV
Procedure details
Retrosynthesis Analysis
AI-Powered Synthesis Planning: Our tool employs the Template_relevance Pistachio, Template_relevance Bkms_metabolic, Template_relevance Pistachio_ringbreaker, Template_relevance Reaxys, Template_relevance Reaxys_biocatalysis model, leveraging a vast database of chemical reactions to predict feasible synthetic routes.
One-Step Synthesis Focus: Specifically designed for one-step synthesis, it provides concise and direct routes for your target compounds, streamlining the synthesis process.
Accurate Predictions: Utilizing the extensive PISTACHIO, BKMS_METABOLIC, PISTACHIO_RINGBREAKER, REAXYS, REAXYS_BIOCATALYSIS database, our tool offers high-accuracy predictions, reflecting the latest in chemical research and data.
Strategy Settings
Precursor scoring | Relevance Heuristic |
---|---|
Min. plausibility | 0.01 |
Model | Template_relevance |
Template Set | Pistachio/Bkms_metabolic/Pistachio_ringbreaker/Reaxys/Reaxys_biocatalysis |
Top-N result to add to graph | 6 |
Feasible Synthetic Routes
Disclaimer and Information on In-Vitro Research Products
Please be aware that all articles and product information presented on BenchChem are intended solely for informational purposes. The products available for purchase on BenchChem are specifically designed for in-vitro studies, which are conducted outside of living organisms. In-vitro studies, derived from the Latin term "in glass," involve experiments performed in controlled laboratory settings using cells or tissues. It is important to note that these products are not categorized as medicines or drugs, and they have not received approval from the FDA for the prevention, treatment, or cure of any medical condition, ailment, or disease. We must emphasize that any form of bodily introduction of these products into humans or animals is strictly prohibited by law. It is essential to adhere to these guidelines to ensure compliance with legal and ethical standards in research and experimentation.