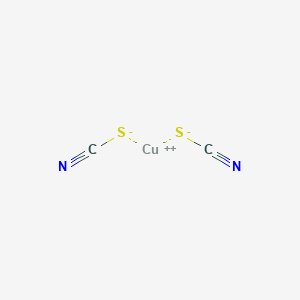
Cuprous thiocyanate
- Click on QUICK INQUIRY to receive a quote from our team of experts.
- With the quality product at a COMPETITIVE price, you can focus more on your research.
Overview
Description
Cuprous thiocyanate, also known as copper(I) thiocyanate, is a coordination polymer with the chemical formula CuSCN. It is an air-stable, white solid that is used as a precursor for the preparation of other thiocyanate salts. This compound is notable for its applications in various fields, including photovoltaics and as a semiconductor .
Mechanism of Action
Target of Action
Cuprous thiocyanate (CuSCN) is a coordination polymer . It is primarily used as a precursor for the preparation of other thiocyanate salts . The compound’s primary targets are the thiocyanate groups (SCN), which are well-known ligands for a variety of molecular complexes .
Mode of Action
CuSCN interacts with its targets through a characteristic tetrahedral coordination geometry . The sulfur end of the SCN- ligand is triply bridging, forming a coordination sphere for copper as CuS3N . This interaction results in the formation of a stable, air-stable, white solid .
Biochemical Pathways
Thiocyanate-degrading microorganisms degrade thiocyanate in an autotrophic manner for energy, while other biodegrading microorganisms use thiocyanate as a carbon or nitrogen source . Two known pathways used by autotrophic microorganisms to degrade thiocyanate are the carbonyl sulfide pathway and the cyanate pathway . These pathways constitute the main biological mechanisms of thiocyanate degradation .
Pharmacokinetics
It is known that the compound is air-stable and has a low solubility in water . This suggests that the compound’s absorption, distribution, metabolism, and excretion (ADME) properties may be influenced by these characteristics.
Result of Action
The result of CuSCN’s action is the formation of a stable, white solid that can be used as a precursor for the preparation of other thiocyanate salts . In addition, CuSCN is a hole conductor and a semiconductor with a wide band gap . It is often used in dye-sensitized solar cells .
Action Environment
The action of CuSCN can be influenced by environmental factors. For example, it has been used as a biocide in antifouling paint formulations . The release of CuSCN into aquatic environments from these paints can affect various non-target organisms and compromise ecosystems . Furthermore, the compound’s action, efficacy, and stability can be influenced by factors such as temperature, pH, and the presence of other chemicals in the environment .
Biochemical Analysis
Biochemical Properties
Cuprous thiocyanate is a P-type semiconductor material, which has the characteristics of abundance, low cost, high carrier mobility, appropriate energy level . It is one of the few known compounds with both high optical transparency and significant P-type electrical conductivity .
Cellular Effects
This compound has been used as a hole transport material in photovoltaics . It acts as a P-type semiconductor and as a solid-state electrolyte . Its hole conductivity is relatively poor but can be improved by various treatments .
Molecular Mechanism
This compound forms from the spontaneous decomposition of black copper (II) thiocyanate, releasing thiocyanogen, especially when heated . It is also formed from copper (II) thiocyanate under water, releasing (among others) thiocyanic acid and the highly poisonous hydrogen cyanide .
Temporal Effects in Laboratory Settings
This compound is an air-stable, white solid . Its hole conductivity is relatively poor but can be improved by various treatments, such as exposure to gaseous chlorine or doping with (SCN) 2 .
Metabolic Pathways
Copper metabolism involves uptake, distribution, sequestration, and excretion, at both the cellular and systemic levels . Cuprous ions are either sequestrated by molecules such as metallothioneins or targeted to utilization pathways by chaperons such as Atox1, Cox17, and CCS .
Transport and Distribution
This compound is transported and distributed within cells and tissues as part of the copper metabolism process . Mammalian enterocytes take in bioavailable copper ions from the diet in a Ctr1-dependent manner . After incorporation, cuprous ions are delivered to ATP7A, which pumps Cu+ from enterocytes into the blood .
Subcellular Localization
Copper ions, which are a component of this compound, are known to be incorporated by Ctr1 in peripheral tissue cells .
Preparation Methods
Synthetic Routes and Reaction Conditions: Cuprous thiocyanate can be synthesized through several methods:
Spontaneous Decomposition: It forms from the spontaneous decomposition of black copper(II) thiocyanate, releasing thiocyanogen, especially when heated.
Aqueous Solution Method: It can be prepared from relatively dilute solutions of copper(II) in water, such as copper(II) sulfate. Sulphurous acid is added to the copper(II) solution, followed by the slow addition of a soluble thiocyanate while stirring.
Thiosulfate Reduction: Alternatively, a thiosulfate solution may be used as a reducing agent to produce this compound.
Industrial Production Methods: Industrial production of this compound typically involves the aqueous solution method due to its simplicity and efficiency. The process is scalable and can be controlled to produce high-purity this compound.
Chemical Reactions Analysis
Cuprous thiocyanate undergoes various chemical reactions, including:
Oxidation: It can be oxidized to form copper(II) thiocyanate.
Reduction: It can be reduced to elemental copper under certain conditions.
Substitution: this compound can participate in substitution reactions where the thiocyanate ligand is replaced by other ligands.
Common Reagents and Conditions:
Oxidizing Agents: Such as hydrogen peroxide or nitric acid.
Reducing Agents: Such as sodium thiosulfate.
Substitution Reagents: Various ligands that can replace the thiocyanate group.
Major Products:
Copper(II) Thiocyanate: Formed through oxidation.
Elemental Copper: Formed through reduction.
Substituted Copper Compounds: Formed through substitution reactions.
Scientific Research Applications
Cuprous thiocyanate has a wide range of scientific research applications:
Chemistry: Used as a precursor for the synthesis of other thiocyanate salts and coordination compounds.
Biology: Investigated for its potential biocidal properties, particularly in antifouling paints.
Medicine: Explored for its antimicrobial properties.
Industry: Utilized in photovoltaics as a hole conductor and semiconductor with a wide band gap. .
Comparison with Similar Compounds
Cuprous thiocyanate can be compared with other similar compounds such as:
Copper(I) Iodide (CuI): Both are used as semiconductors, but this compound has a wider band gap and is more stable in air.
Copper(I) Cyanide (CuCN): Similar in structure but differs in its reactivity and applications.
Ammonium Thiocyanate (NH4SCN): Used in different applications, primarily as a reagent in chemical synthesis.
Uniqueness: this compound is unique due to its combination of stability, wide band gap, and versatility in applications ranging from photovoltaics to antifouling paints .
Properties
CAS No. |
1111-67-7 |
---|---|
Molecular Formula |
CHNS.Cu CHCuNS |
Molecular Weight |
122.64 g/mol |
IUPAC Name |
copper;thiocyanic acid |
InChI |
InChI=1S/CHNS.Cu/c2-1-3;/h3H; |
InChI Key |
WEKJRGBVEBCTRF-UHFFFAOYSA-N |
SMILES |
C(#N)[S-].[Cu+] |
Canonical SMILES |
C(#N)S.[Cu] |
1111-67-7 | |
physical_description |
Dry Powder White to yellow solid; [Merck Index] White-gray odorless solid; [ECHA - Proposal for Harmonised Classification and Labelling] Powder; [Sigma-Aldrich MSDS] |
Pictograms |
Environmental Hazard |
Synonyms |
copper thiocyanate cuprous thiocyanate CuSCN |
Origin of Product |
United States |
Retrosynthesis Analysis
AI-Powered Synthesis Planning: Our tool employs the Template_relevance Pistachio, Template_relevance Bkms_metabolic, Template_relevance Pistachio_ringbreaker, Template_relevance Reaxys, Template_relevance Reaxys_biocatalysis model, leveraging a vast database of chemical reactions to predict feasible synthetic routes.
One-Step Synthesis Focus: Specifically designed for one-step synthesis, it provides concise and direct routes for your target compounds, streamlining the synthesis process.
Accurate Predictions: Utilizing the extensive PISTACHIO, BKMS_METABOLIC, PISTACHIO_RINGBREAKER, REAXYS, REAXYS_BIOCATALYSIS database, our tool offers high-accuracy predictions, reflecting the latest in chemical research and data.
Strategy Settings
Precursor scoring | Relevance Heuristic |
---|---|
Min. plausibility | 0.01 |
Model | Template_relevance |
Template Set | Pistachio/Bkms_metabolic/Pistachio_ringbreaker/Reaxys/Reaxys_biocatalysis |
Top-N result to add to graph | 6 |
Feasible Synthetic Routes
Disclaimer and Information on In-Vitro Research Products
Please be aware that all articles and product information presented on BenchChem are intended solely for informational purposes. The products available for purchase on BenchChem are specifically designed for in-vitro studies, which are conducted outside of living organisms. In-vitro studies, derived from the Latin term "in glass," involve experiments performed in controlled laboratory settings using cells or tissues. It is important to note that these products are not categorized as medicines or drugs, and they have not received approval from the FDA for the prevention, treatment, or cure of any medical condition, ailment, or disease. We must emphasize that any form of bodily introduction of these products into humans or animals is strictly prohibited by law. It is essential to adhere to these guidelines to ensure compliance with legal and ethical standards in research and experimentation.