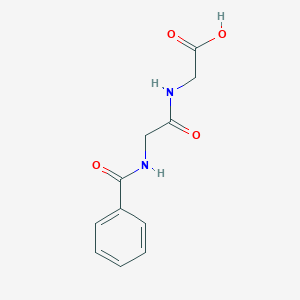
Benzoylglycylglycine
Overview
Description
Synthesis Analysis
The synthesis of organotin derivatives of N-benzoylglycylglycine involves characterizing these compounds using infrared, 1H, 13C NMR, and 119Sn Mossbauer spectroscopy. Diorganotin derivatives are identified as dimer distannoxanes with a ladder-type structure, where tin atoms are five-coordinated, and N-benzoylglycylglycine acts as a unidentate or bridging bidentate ligand through the carboxylate group. Triorganotin derivatives propose a polymeric structure where N-benzoylglycylglycine bridges planar SnC3 units through the carboxylate group (Ruisi & Giudice, 1991).
Molecular Structure Analysis
Studies on intermolecular complex formation, such as the crystal structure of cytosine-N-benzoylglycine complex monohydrate, reveal the intermolecular interactions and structural details of N-benzoylglycylglycine. The crystal structure demonstrates how cytosinyl cation and N-benzoylglycyl anion are joined by two NH···O hydrogen bonds, suggesting complex formation possibilities in vivo (Tamura et al., 1972).
Chemical Reactions and Properties
The reaction of N-benzoyl-2-bromoglycine methyl ester with deprotonated nitroalkanes to synthesize β-nitro and α,β-dehydro amino acid derivatives showcases the chemical reactivity and potential transformations of N-benzoylglycylglycine or its derivatives (Burgess & Easton, 1988).
Physical Properties Analysis
The characterization of organotin derivatives of N-benzoylglycylglycine, including their synthesis and IR, 1H NMR spectral studies, provides insight into the physical properties of these compounds. These studies indicate the formation of penta-coordinate trigonal bipyramidal structure of tin(IV) with chelating bidentate carboxylate groups in solid and solution states, revealing important aspects of the physical properties of N-benzoylglycylglycine complexes (Sandhu & Kaur, 1990).
Chemical Properties Analysis
The chemical properties of N-benzoylglycylglycine can be inferred from studies on its derivatives and reactions. For instance, the synthesis and structural characterization of N-meta-ferrocenyl benzoyl dipeptide esters and their in vitro anti-cancer activity highlight the versatility and chemical reactivity of N-benzoylglycylglycine derivatives, indicating their potential applications in medicinal chemistry and material science (Goel et al., 2007).
Scientific Research Applications
Organotin Derivatives Synthesis : Di- and tri-organotin derivatives of N-benzoylglycylglycine were synthesized and characterized, highlighting its role in forming compounds with potential applications in organometallic chemistry (Ruisi & Giudice, 1991).
Triorganotin(IV) Complexes : N-benzoylglycylglycine was used to prepare triorganotin(IV) derivatives, providing insights into the structural aspects of these complexes which are valuable in organometallic chemistry (Sandhu & Kaur, 1990).
Aldose Reductase Inhibitory Activity : Synthesized N-benzoylglycines showed potential as aldose reductase inhibitors, which are significant in the context of diabetes and its complications (Deruiter et al., 1989).
Radiopharmaceutical Imaging Agents : Studies have focused on the use of N-benzoylglycylglycine derivatives in the preparation of radiopharmaceutical imaging agents, particularly for renal studies (Mobashar et al., 1991).
Neurodevelopmental Outcomes in Nonketotic Hyperglycinemia : Research has also explored the effectiveness of sodium benzoate, which conjugates with glycine, in treating nonketotic hyperglycinemia, highlighting the metabolic role of glycine derivatives (Bjoraker et al., 2016).
Gold Nanoparticles Conjugation : Benzoyl-protected mercaptoacetyltriglycine, a derivative of N-benzoylglycylglycine, was used as a capping agent for gold nanoparticles, showcasing its potential in nanotechnology (Estévez-Hernández et al., 2010).
Fluorogenic Substrate in Enzyme Assays : It has been applied as a fluorogenic substrate in enzyme assays, demonstrating its utility in biochemical research (Carmel & Yaron, 1978).
Sentinel Node Detection in Medical Imaging : The compound has been studied for its use in receptor-binding radiopharmaceuticals for sentinel node detection, indicating its application in medical imaging (Vera et al., 2001).
Intermolecular Complex Formation Studies : Its intermolecular complexes with other chemical entities have been studied, providing insights into its potential in crystallography and molecular interaction studies (Tamura et al., 1972).
Biological Activity Synthesis : N-benzoyl cyanoacetylhydrazine, related to N-benzoylglycylglycine, has been used in synthesizing heterocyclic compounds with potential biological activity, highlighting its versatility in medicinal chemistry (Mohareb et al., 2007).
Future Directions
properties
IUPAC Name |
2-[(2-benzamidoacetyl)amino]acetic acid | |
---|---|---|
Source | PubChem | |
URL | https://pubchem.ncbi.nlm.nih.gov | |
Description | Data deposited in or computed by PubChem | |
InChI |
InChI=1S/C11H12N2O4/c14-9(12-7-10(15)16)6-13-11(17)8-4-2-1-3-5-8/h1-5H,6-7H2,(H,12,14)(H,13,17)(H,15,16) | |
Source | PubChem | |
URL | https://pubchem.ncbi.nlm.nih.gov | |
Description | Data deposited in or computed by PubChem | |
InChI Key |
FWYPSSZPXQVFRB-UHFFFAOYSA-N | |
Source | PubChem | |
URL | https://pubchem.ncbi.nlm.nih.gov | |
Description | Data deposited in or computed by PubChem | |
Canonical SMILES |
C1=CC=C(C=C1)C(=O)NCC(=O)NCC(=O)O | |
Source | PubChem | |
URL | https://pubchem.ncbi.nlm.nih.gov | |
Description | Data deposited in or computed by PubChem | |
Molecular Formula |
C11H12N2O4 | |
Source | PubChem | |
URL | https://pubchem.ncbi.nlm.nih.gov | |
Description | Data deposited in or computed by PubChem | |
DSSTOX Substance ID |
DTXSID70150748 | |
Record name | N-Hippurylglycine | |
Source | EPA DSSTox | |
URL | https://comptox.epa.gov/dashboard/DTXSID70150748 | |
Description | DSSTox provides a high quality public chemistry resource for supporting improved predictive toxicology. | |
Molecular Weight |
236.22 g/mol | |
Source | PubChem | |
URL | https://pubchem.ncbi.nlm.nih.gov | |
Description | Data deposited in or computed by PubChem | |
CAS RN |
1145-32-0 | |
Record name | N-Hippurylglycine | |
Source | ChemIDplus | |
URL | https://pubchem.ncbi.nlm.nih.gov/substance/?source=chemidplus&sourceid=0001145320 | |
Description | ChemIDplus is a free, web search system that provides access to the structure and nomenclature authority files used for the identification of chemical substances cited in National Library of Medicine (NLM) databases, including the TOXNET system. | |
Record name | Benzoylglycylglycine | |
Source | DTP/NCI | |
URL | https://dtp.cancer.gov/dtpstandard/servlet/dwindex?searchtype=NSC&outputformat=html&searchlist=89164 | |
Description | The NCI Development Therapeutics Program (DTP) provides services and resources to the academic and private-sector research communities worldwide to facilitate the discovery and development of new cancer therapeutic agents. | |
Explanation | Unless otherwise indicated, all text within NCI products is free of copyright and may be reused without our permission. Credit the National Cancer Institute as the source. | |
Record name | N-Hippurylglycine | |
Source | EPA DSSTox | |
URL | https://comptox.epa.gov/dashboard/DTXSID70150748 | |
Description | DSSTox provides a high quality public chemistry resource for supporting improved predictive toxicology. | |
Retrosynthesis Analysis
AI-Powered Synthesis Planning: Our tool employs the Template_relevance Pistachio, Template_relevance Bkms_metabolic, Template_relevance Pistachio_ringbreaker, Template_relevance Reaxys, Template_relevance Reaxys_biocatalysis model, leveraging a vast database of chemical reactions to predict feasible synthetic routes.
One-Step Synthesis Focus: Specifically designed for one-step synthesis, it provides concise and direct routes for your target compounds, streamlining the synthesis process.
Accurate Predictions: Utilizing the extensive PISTACHIO, BKMS_METABOLIC, PISTACHIO_RINGBREAKER, REAXYS, REAXYS_BIOCATALYSIS database, our tool offers high-accuracy predictions, reflecting the latest in chemical research and data.
Strategy Settings
Precursor scoring | Relevance Heuristic |
---|---|
Min. plausibility | 0.01 |
Model | Template_relevance |
Template Set | Pistachio/Bkms_metabolic/Pistachio_ringbreaker/Reaxys/Reaxys_biocatalysis |
Top-N result to add to graph | 6 |
Feasible Synthetic Routes
Q & A
Q1: How does Benzoylglycylglycine interact with organotin compounds, and what are the structural implications?
A1: this compound (HBzGlyGly) acts as a ligand, coordinating with organotin compounds through its carboxylate group. [, ] In the case of diorganotin derivatives, HBzGlyGly forms dimeric distannoxane structures ([R2SnBzGlyGly]2O)2 (where R = CH3, n-C4H9, n-C8H17). These structures adopt a ladder-like configuration where the tin atoms are five-coordinated. Interestingly, HBzGlyGly exhibits structural flexibility, acting as either a unidentate or bridging bidentate ligand. [] For triorganotin derivatives (R3SnBzGlyGly, where R = CH3, n-C4H9), a polymeric structure is proposed, with HBzGlyGly bridging planar SnC3 units via the carboxylate group. []
Q2: What spectroscopic techniques are valuable for characterizing organotin derivatives of this compound?
A2: Several spectroscopic techniques prove invaluable for characterizing these organotin derivatives. Infrared (IR) spectroscopy helps identify the coordination mode of HBzGlyGly by analyzing shifts in carboxylate group vibrations. Nuclear magnetic resonance (NMR) spectroscopy, specifically 1H, 13C, and 119Sn NMR, provides insights into the structure and bonding environment within the complexes. [] Additionally, 119Sn Mössbauer spectroscopy offers information about the tin atom's oxidation state and coordination geometry. [] These complementary techniques, when combined, paint a comprehensive picture of the structure and properties of these organometallic compounds.
Q3: Beyond organotin compounds, are there other potential applications for this compound in materials science or related fields?
A3: While the provided research focuses on organotin interactions, the structural features of this compound hint at broader applications. The presence of both peptide and carboxylate functionalities suggests potential as a building block for supramolecular assemblies or as a ligand for other metals. Furthermore, its ability to form polymeric structures with triorganotin compounds [] opens possibilities for designing novel metal-organic frameworks (MOFs) or coordination polymers. Further research exploring these avenues could reveal exciting new applications for this versatile molecule.
Disclaimer and Information on In-Vitro Research Products
Please be aware that all articles and product information presented on BenchChem are intended solely for informational purposes. The products available for purchase on BenchChem are specifically designed for in-vitro studies, which are conducted outside of living organisms. In-vitro studies, derived from the Latin term "in glass," involve experiments performed in controlled laboratory settings using cells or tissues. It is important to note that these products are not categorized as medicines or drugs, and they have not received approval from the FDA for the prevention, treatment, or cure of any medical condition, ailment, or disease. We must emphasize that any form of bodily introduction of these products into humans or animals is strictly prohibited by law. It is essential to adhere to these guidelines to ensure compliance with legal and ethical standards in research and experimentation.