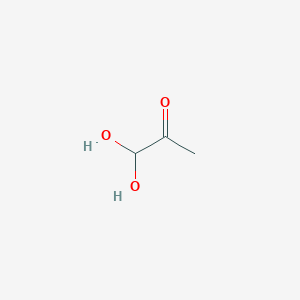
1,1-Dihydroxypropan-2-one
Overview
Description
1,1-Dihydroxypropan-2-one, also known as dihydroxyacetone (DHA), is a small molecule that has gained significant attention in the scientific community due to its unique properties. DHA is a colorless, odorless, and water-soluble compound that is commonly used in the cosmetic industry as a self-tanning agent. However, recent studies have shown that DHA has potential applications in various fields, including scientific research.
Scientific Research Applications
Membrane Distillation in Separation Processes
Wang Shichang (2008) explored the separation of 1,2-dihydroxypropane from its aqueous solution using vacuum membrane distillation. The study found that permeation flux increased with vacuum degree, feed flow, and feed temperature, while retention rate decreased under similar conditions, demonstrating the feasibility of this method for separating 1,2-dihydroxypropane (Wang Shichang, 2008).
Hydrothermal Degradation and Kinetics
Bonn, Rinderer, and Bobleter (1985) studied the equilibrium of 1,3-dihydroxy-2-propanone and its dehydration reaction to methylglyoxal under hydrothermal conditions. This research provides insights into the reaction mechanism for these C-3 compounds (Bonn, Rinderer, & Bobleter, 1985).
Catalysis and Enantioselective Hydrogenation
Cesarotti et al. (1993) synthesized various 3-O-substituted 1,3-dihydroxypropan-2-ones, reducing them to optically active glycerols using chiral Ru complexes. This research contributes to understanding the role of 1,3-dihydroxypropan-2-ones in asymmetric synthesis (Cesarotti, Antognazza, Pallavicini, & Villa, 1993).
Synthesis of Derivatives
Kubota et al. (1977) synthesized new 1,3-dithiane-2-thione derivatives from bismesylates of substituted 1,3-dihydroxypropanes. The findings are important for understanding the synthesis and properties of these derivatives (Kubota, Taniguchi, Eto, & Maekawa, 1977).
Zeolite Catalysis in Alcohol Dehydration
Jacobs, Tielen, and Uytterhoeven (1977) investigated alcohol dehydration over alkali cation-exchanged zeolites X and Y. The study provides valuable information on the catalytic processes and reaction mechanisms involved (Jacobs, Tielen, & Uytterhoeven, 1977).
Cooperative Transformation in Catalysis
Dai et al. (2015) prepared a CuNiAlOx catalyst for transforming biomass-based alcohols, such as glycerol, directly into 1,3-dihydroxypropan-2-one. This study is significant for understanding catalytic processes in biomass conversion (Dai, Cui, Yuan, Deng, & Shi, 2015).
properties
CAS RN |
1186-47-6 |
---|---|
Product Name |
1,1-Dihydroxypropan-2-one |
Molecular Formula |
C3H6O3 |
Molecular Weight |
90.08 g/mol |
IUPAC Name |
1,1-dihydroxypropan-2-one |
InChI |
InChI=1S/C3H6O3/c1-2(4)3(5)6/h3,5-6H,1H3 |
InChI Key |
UOQFZGVGGMHGEE-UHFFFAOYSA-N |
SMILES |
CC(=O)C(O)O |
Canonical SMILES |
CC(=O)C(O)O |
synonyms |
methylglyoxal hydrate |
Origin of Product |
United States |
Synthesis routes and methods I
Procedure details
Synthesis routes and methods II
Procedure details
Retrosynthesis Analysis
AI-Powered Synthesis Planning: Our tool employs the Template_relevance Pistachio, Template_relevance Bkms_metabolic, Template_relevance Pistachio_ringbreaker, Template_relevance Reaxys, Template_relevance Reaxys_biocatalysis model, leveraging a vast database of chemical reactions to predict feasible synthetic routes.
One-Step Synthesis Focus: Specifically designed for one-step synthesis, it provides concise and direct routes for your target compounds, streamlining the synthesis process.
Accurate Predictions: Utilizing the extensive PISTACHIO, BKMS_METABOLIC, PISTACHIO_RINGBREAKER, REAXYS, REAXYS_BIOCATALYSIS database, our tool offers high-accuracy predictions, reflecting the latest in chemical research and data.
Strategy Settings
Precursor scoring | Relevance Heuristic |
---|---|
Min. plausibility | 0.01 |
Model | Template_relevance |
Template Set | Pistachio/Bkms_metabolic/Pistachio_ringbreaker/Reaxys/Reaxys_biocatalysis |
Top-N result to add to graph | 6 |
Feasible Synthetic Routes
Disclaimer and Information on In-Vitro Research Products
Please be aware that all articles and product information presented on BenchChem are intended solely for informational purposes. The products available for purchase on BenchChem are specifically designed for in-vitro studies, which are conducted outside of living organisms. In-vitro studies, derived from the Latin term "in glass," involve experiments performed in controlled laboratory settings using cells or tissues. It is important to note that these products are not categorized as medicines or drugs, and they have not received approval from the FDA for the prevention, treatment, or cure of any medical condition, ailment, or disease. We must emphasize that any form of bodily introduction of these products into humans or animals is strictly prohibited by law. It is essential to adhere to these guidelines to ensure compliance with legal and ethical standards in research and experimentation.