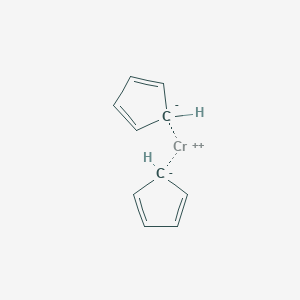
Chromocene
Overview
Description
Chromocene, also known as this compound, is an organochromium compound with the formula [Cr(C₅H₅)₂]. It is a member of the metallocene family, which includes compounds like ferrocene and nickelocene. This compound is characterized by its dark red crystalline appearance and its ability to sublime in a vacuum .
Mechanism of Action
Target of Action
Bis(cyclopentadienyl)chromium(II) primarily targets chemical reactions as a catalyst . It is used in stereoselective pinacol-type cross-coupling reactions, thermal homo-polymerization and co-polymerization reactions, and Nozaki-Hiyama-Kishi reactions (additions of organic halides to aldehydes) .
Mode of Action
As a catalyst, Bis(cyclopentadienyl)chromium(II) facilitates chemical reactions without being consumed in the process . It interacts with its targets by lowering the activation energy of the reaction, enabling the reaction to proceed more efficiently .
Biochemical Pathways
The exact biochemical pathways affected by Bis(cyclopentadienyl)chromium(II) are dependent on the specific reactions it catalyzes. For instance, in stereoselective pinacol-type cross-coupling reactions, it can influence the formation of certain organic compounds . .
Result of Action
The result of Bis(cyclopentadienyl)chromium(II)'s action is the successful facilitation of the chemical reactions it catalyzes . This can lead to the efficient production of desired compounds in these reactions .
Preparation Methods
Synthetic Routes and Reaction Conditions
One common method for preparing bis(cyclopentadienyl)chromium(II) involves the reaction of chromium(II) chloride with sodium cyclopentadienide in tetrahydrofuran. The reaction can be represented as follows: [ \text{CrCl}_2 + 2 \text{NaC}_5\text{H}_5 \rightarrow \text{Cr(C}_5\text{H}_5\text{)}_2 + 2 \text{NaCl} ] Another method involves the reduction of chromium(III) chloride with sodium cyclopentadienide .
Chemical Reactions Analysis
Types of Reactions
Chromocene undergoes various types of chemical reactions, including:
Oxidation: It can be oxidized to form chromium(III) compounds.
Reduction: It can act as a reducing agent in certain reactions.
Substitution: The cyclopentadienyl ligands can be displaced by other ligands under specific conditions.
Common Reagents and Conditions
Oxidation: Common oxidizing agents include oxygen and halogens.
Reduction: Reducing agents such as lithium aluminum hydride can be used.
Substitution: Ligand exchange reactions often require elevated temperatures and specific solvents.
Major Products
Oxidation: Chromium(III) compounds.
Reduction: Chromium(0) compounds.
Substitution: Various substituted this compound derivatives.
Scientific Research Applications
Chromocene has several applications in scientific research:
Medicine: Research is ongoing to investigate its potential therapeutic uses.
Industry: It is used in the production of certain polymers and as a catalyst in various industrial processes.
Comparison with Similar Compounds
Similar Compounds
Ferrocene: [Fe(C₅H₅)₂]
Nickelocene: [Ni(C₅H₅)₂]
Bis(benzene)chromium: [Cr(C₆H₆)₂]
Bis(cyclopentadienyl)cobalt(II): [Co(C₅H₅)₂]
Bis(cyclopentadienyl)nickel(II): [Ni(C₅H₅)₂]
Uniqueness
Chromocene is unique due to its specific redox properties and its ability to act as a catalyst in a variety of chemical reactions. Its structure and bonding also differ from other metallocenes, making it a valuable compound in both research and industrial applications .
Properties
CAS No. |
1271-24-5 |
---|---|
Molecular Formula |
C10H10Cr |
Molecular Weight |
182.18 g/mol |
IUPAC Name |
chromium(2+);cyclopenta-1,3-diene |
InChI |
InChI=1S/2C5H5.Cr/c2*1-2-4-5-3-1;/h2*1-3H,4H2;/q2*-1;+2 |
InChI Key |
WQGIIPSPIWDBHR-UHFFFAOYSA-N |
SMILES |
[CH-]1C=CC=C1.[CH-]1C=CC=C1.[Cr+2] |
Canonical SMILES |
C1C=CC=[C-]1.C1C=CC=[C-]1.[Cr+2] |
boiling_point |
167 to 194 °F at 760 mmHg under vacuum (sublimes) (NTP, 1992) |
melting_point |
343 °F (NTP, 1992) |
Key on ui other cas no. |
1271-24-5 |
physical_description |
Bis(cyclopentadienyl)chromium appears as red needles or reddish-purple solid. (NTP, 1992) |
solubility |
Decomposes (NTP, 1992) |
Origin of Product |
United States |
Synthesis routes and methods
Procedure details
Retrosynthesis Analysis
AI-Powered Synthesis Planning: Our tool employs the Template_relevance Pistachio, Template_relevance Bkms_metabolic, Template_relevance Pistachio_ringbreaker, Template_relevance Reaxys, Template_relevance Reaxys_biocatalysis model, leveraging a vast database of chemical reactions to predict feasible synthetic routes.
One-Step Synthesis Focus: Specifically designed for one-step synthesis, it provides concise and direct routes for your target compounds, streamlining the synthesis process.
Accurate Predictions: Utilizing the extensive PISTACHIO, BKMS_METABOLIC, PISTACHIO_RINGBREAKER, REAXYS, REAXYS_BIOCATALYSIS database, our tool offers high-accuracy predictions, reflecting the latest in chemical research and data.
Strategy Settings
Precursor scoring | Relevance Heuristic |
---|---|
Min. plausibility | 0.01 |
Model | Template_relevance |
Template Set | Pistachio/Bkms_metabolic/Pistachio_ringbreaker/Reaxys/Reaxys_biocatalysis |
Top-N result to add to graph | 6 |
Feasible Synthetic Routes
Q1: How does Bis(cyclopentadienyl)chromium(II) impact the thermo-oxidative stability of HDPE compared to traditional Ziegler-Natta catalysts?
A: While Bis(cyclopentadienyl)chromium(II) effectively catalyzes HDPE production, it can negatively affect the polymer's long-term stability. Research indicates that HDPE produced using Bis(cyclopentadienyl)chromium(II) exhibits lower intrinsic thermo-oxidative stability compared to HDPE synthesized with traditional Ziegler-Natta catalysts []. This difference arises from the nature of the catalyst residues left within the polymer matrix.
Q2: How does the silica support used with Bis(cyclopentadienyl)chromium(II) contribute to the pro-oxidative activity in HDPE?
A: During gas-phase polymerization, the silica support used with Bis(cyclopentadienyl)chromium(II) undergoes significant changes. Scanning electron microscopy reveals that the silica support shatters into submicron fragments, which then become dispersed throughout the polymer particles []. Upon exposure to air, the Bis(cyclopentadienyl)chromium(II) on these fragments oxidizes to a trivalent chromium species (Cr(III)), confirmed through UV/visible spectrophotometry and diffuse reflectance Fourier transform infrared (FTIR) spectroscopy []. This Cr(III) species, bound to the high surface area silica fragments, acts as a potent pro-oxidant, further contributing to the decreased thermo-oxidative stability of the resulting HDPE.
Disclaimer and Information on In-Vitro Research Products
Please be aware that all articles and product information presented on BenchChem are intended solely for informational purposes. The products available for purchase on BenchChem are specifically designed for in-vitro studies, which are conducted outside of living organisms. In-vitro studies, derived from the Latin term "in glass," involve experiments performed in controlled laboratory settings using cells or tissues. It is important to note that these products are not categorized as medicines or drugs, and they have not received approval from the FDA for the prevention, treatment, or cure of any medical condition, ailment, or disease. We must emphasize that any form of bodily introduction of these products into humans or animals is strictly prohibited by law. It is essential to adhere to these guidelines to ensure compliance with legal and ethical standards in research and experimentation.