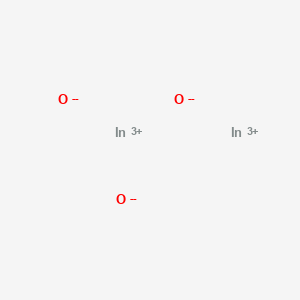
Indium oxide
Overview
Description
Indium oxide, also known as indium trioxide, is a chemical compound with the formula In₂O₃. It is an amphoteric oxide of indium, meaning it can react with both acids and bases. This compound is typically found as a yellowish-green, odorless crystal and is insoluble in water. It has a cubic crystal structure and is known for its high melting point of approximately 1,910°C .
Mechanism of Action
Target of Action
Indium oxide (In2O3) is a chemical compound that primarily targets semiconductor materials and microelectronic devices . It is used in a variety of applications, including solar cells, flat-panel display sensors, transparent conductors, and architectural glasses . The compound’s role is to enhance the performance of these devices, often by improving their electrical conductivity and optical transmittance .
Mode of Action
This compound interacts with its targets by forming ionic and partial covalent bonds with oxygen atoms . This interaction results in a change in the electronic and optical properties of the material. For instance, it can alter the optical metallic nature of cubic and orthorhombic structures and the semiconducting nature of trigonal structures .
Biochemical Pathways
This process involves a complex matrix of parameters, and some phases are only obtained through controlled phase transformations .
Pharmacokinetics
It’s worth noting that this compound isinsoluble in water but soluble in acids . This property can impact its distribution and availability in various environments.
Result of Action
The action of this compound results in materials with enhanced electrical and optical properties . For example, thin films of this compound doped with Zn2+ are highly conductive and even superconductive at liquid helium temperatures . Furthermore, this compound can form a layer on a silicon substrate, a method useful for the manufacture of solar cells .
Action Environment
The action of this compound can be influenced by environmental factors. For instance, the formation of oxygen vacancies in this compound, which is crucial for its photothermal catalytic performance, can be observed under certain environmental conditions . Additionally, the synthesis of this compound phases involves maneuvering in a complex matrix of process parameters , suggesting that the compound’s action, efficacy, and stability can be influenced by the specific conditions of its environment.
Biochemical Analysis
Biochemical Properties
Indium oxide is known for its role as a photothermal catalyst . It interacts with various biomolecules, particularly in the context of oxygen vacancies . These vacancies act as active sites and are responsible for the remarkable photothermal activity .
Molecular Mechanism
This compound exerts its effects at the molecular level through its oxygen vacancies . These vacancies can interact with biomolecules, potentially influencing enzyme activity and gene expression .
Temporal Effects in Laboratory Settings
In laboratory settings, the effects of this compound can change over time . For instance, the compound’s photothermal activity can be influenced by temperature .
Transport and Distribution
It’s known that the compound can interact with oxygen vacancies, which may influence its localization or accumulation .
Preparation Methods
Synthetic Routes and Reaction Conditions: Indium oxide can be synthesized through various methods. One common laboratory method involves heating indium hydroxide, nitrate, carbonate, or sulfate at high temperatures. For instance, indium hydroxide can be thermally decomposed to produce this compound .
Industrial Production Methods: In industrial settings, this compound is often produced by sputtering indium targets in an argon-oxygen atmosphere. This method is particularly useful for creating thin films of this compound, which are used in various electronic applications .
Chemical Reactions Analysis
Types of Reactions: Indium oxide undergoes several types of chemical reactions, including oxidation, reduction, and substitution reactions.
Common Reagents and Conditions:
Oxidation: this compound can be further oxidized to form higher oxidation state compounds under specific conditions.
Reduction: It can be reduced by hydrogen gas at high temperatures to produce metallic indium and water.
Substitution: this compound can react with various acids to form indium salts and water.
Major Products:
Oxidation: Higher oxidation state compounds of indium.
Reduction: Metallic indium and water.
Substitution: Indium salts and water.
Scientific Research Applications
Indium oxide has a wide range of applications in scientific research and industry:
Comparison with Similar Compounds
- Tin oxide (SnO₂)
- Zinc oxide (ZnO)
- Titanium dioxide (TiO₂)
Indium oxide stands out due to its unique combination of electrical conductivity, optical transparency, and high-temperature stability, making it a valuable material in various advanced technological applications.
Properties
IUPAC Name |
indium(3+);oxygen(2-) | |
---|---|---|
Source | PubChem | |
URL | https://pubchem.ncbi.nlm.nih.gov | |
Description | Data deposited in or computed by PubChem | |
InChI |
InChI=1S/2In.3O/q2*+3;3*-2 | |
Source | PubChem | |
URL | https://pubchem.ncbi.nlm.nih.gov | |
Description | Data deposited in or computed by PubChem | |
InChI Key |
PJXISJQVUVHSOJ-UHFFFAOYSA-N | |
Source | PubChem | |
URL | https://pubchem.ncbi.nlm.nih.gov | |
Description | Data deposited in or computed by PubChem | |
Canonical SMILES |
[O-2].[O-2].[O-2].[In+3].[In+3] | |
Source | PubChem | |
URL | https://pubchem.ncbi.nlm.nih.gov | |
Description | Data deposited in or computed by PubChem | |
Molecular Formula |
In2O3 | |
Record name | indium(III) oxide | |
Source | Wikipedia | |
URL | https://en.wikipedia.org/wiki/Indium(III)_oxide | |
Description | Chemical information link to Wikipedia. | |
Source | PubChem | |
URL | https://pubchem.ncbi.nlm.nih.gov | |
Description | Data deposited in or computed by PubChem | |
DSSTOX Substance ID |
DTXSID40893857 | |
Record name | Indium(3+) oxide | |
Source | EPA DSSTox | |
URL | https://comptox.epa.gov/dashboard/DTXSID40893857 | |
Description | DSSTox provides a high quality public chemistry resource for supporting improved predictive toxicology. | |
Molecular Weight |
277.63 g/mol | |
Source | PubChem | |
URL | https://pubchem.ncbi.nlm.nih.gov | |
Description | Data deposited in or computed by PubChem | |
Physical Description |
White to pale yellow solid; [Merck Index] Yellow odorless powder; [MSDSonline] | |
Record name | Indium oxide | |
Source | Haz-Map, Information on Hazardous Chemicals and Occupational Diseases | |
URL | https://haz-map.com/Agents/9308 | |
Description | Haz-Map® is an occupational health database designed for health and safety professionals and for consumers seeking information about the adverse effects of workplace exposures to chemical and biological agents. | |
Explanation | Copyright (c) 2022 Haz-Map(R). All rights reserved. Unless otherwise indicated, all materials from Haz-Map are copyrighted by Haz-Map(R). No part of these materials, either text or image may be used for any purpose other than for personal use. Therefore, reproduction, modification, storage in a retrieval system or retransmission, in any form or by any means, electronic, mechanical or otherwise, for reasons other than personal use, is strictly prohibited without prior written permission. | |
CAS No. |
1312-43-2, 12672-71-8 | |
Record name | Indium oxide | |
Source | ChemIDplus | |
URL | https://pubchem.ncbi.nlm.nih.gov/substance/?source=chemidplus&sourceid=0001312432 | |
Description | ChemIDplus is a free, web search system that provides access to the structure and nomenclature authority files used for the identification of chemical substances cited in National Library of Medicine (NLM) databases, including the TOXNET system. | |
Record name | Indium oxide | |
Source | ChemIDplus | |
URL | https://pubchem.ncbi.nlm.nih.gov/substance/?source=chemidplus&sourceid=0012672718 | |
Description | ChemIDplus is a free, web search system that provides access to the structure and nomenclature authority files used for the identification of chemical substances cited in National Library of Medicine (NLM) databases, including the TOXNET system. | |
Record name | Indium oxide (In2O3) | |
Source | EPA Chemicals under the TSCA | |
URL | https://www.epa.gov/chemicals-under-tsca | |
Description | EPA Chemicals under the Toxic Substances Control Act (TSCA) collection contains information on chemicals and their regulations under TSCA, including non-confidential content from the TSCA Chemical Substance Inventory and Chemical Data Reporting. | |
Record name | Indium(3+) oxide | |
Source | EPA DSSTox | |
URL | https://comptox.epa.gov/dashboard/DTXSID40893857 | |
Description | DSSTox provides a high quality public chemistry resource for supporting improved predictive toxicology. | |
Record name | Diindium trioxide | |
Source | European Chemicals Agency (ECHA) | |
URL | https://echa.europa.eu/substance-information/-/substanceinfo/100.013.813 | |
Description | The European Chemicals Agency (ECHA) is an agency of the European Union which is the driving force among regulatory authorities in implementing the EU's groundbreaking chemicals legislation for the benefit of human health and the environment as well as for innovation and competitiveness. | |
Explanation | Use of the information, documents and data from the ECHA website is subject to the terms and conditions of this Legal Notice, and subject to other binding limitations provided for under applicable law, the information, documents and data made available on the ECHA website may be reproduced, distributed and/or used, totally or in part, for non-commercial purposes provided that ECHA is acknowledged as the source: "Source: European Chemicals Agency, http://echa.europa.eu/". Such acknowledgement must be included in each copy of the material. ECHA permits and encourages organisations and individuals to create links to the ECHA website under the following cumulative conditions: Links can only be made to webpages that provide a link to the Legal Notice page. | |
Record name | Indium oxide | |
Source | European Chemicals Agency (ECHA) | |
URL | https://echa.europa.eu/substance-information/-/substanceinfo/100.032.501 | |
Description | The European Chemicals Agency (ECHA) is an agency of the European Union which is the driving force among regulatory authorities in implementing the EU's groundbreaking chemicals legislation for the benefit of human health and the environment as well as for innovation and competitiveness. | |
Explanation | Use of the information, documents and data from the ECHA website is subject to the terms and conditions of this Legal Notice, and subject to other binding limitations provided for under applicable law, the information, documents and data made available on the ECHA website may be reproduced, distributed and/or used, totally or in part, for non-commercial purposes provided that ECHA is acknowledged as the source: "Source: European Chemicals Agency, http://echa.europa.eu/". Such acknowledgement must be included in each copy of the material. ECHA permits and encourages organisations and individuals to create links to the ECHA website under the following cumulative conditions: Links can only be made to webpages that provide a link to the Legal Notice page. | |
Record name | INDIUM OXIDE | |
Source | FDA Global Substance Registration System (GSRS) | |
URL | https://gsrs.ncats.nih.gov/ginas/app/beta/substances/4OO9KME22D | |
Description | The FDA Global Substance Registration System (GSRS) enables the efficient and accurate exchange of information on what substances are in regulated products. Instead of relying on names, which vary across regulatory domains, countries, and regions, the GSRS knowledge base makes it possible for substances to be defined by standardized, scientific descriptions. | |
Explanation | Unless otherwise noted, the contents of the FDA website (www.fda.gov), both text and graphics, are not copyrighted. They are in the public domain and may be republished, reprinted and otherwise used freely by anyone without the need to obtain permission from FDA. Credit to the U.S. Food and Drug Administration as the source is appreciated but not required. | |
Q1: What is the molecular formula and weight of indium oxide?
A1: this compound has the molecular formula In2O3 and a molecular weight of 277.64 g/mol.
Q2: What spectroscopic data is available for characterizing this compound?
A2: Common spectroscopic techniques for In2O3 characterization include:* X-ray diffraction (XRD): Determines the crystal structure and phase purity of In2O3 films and powders [, , ].* Raman spectroscopy: Identifies characteristic vibrational modes, confirming the cubic structure of In2O3 thin films [].* X-ray photoelectron spectroscopy (XPS): Analyzes the chemical composition and bonding states of elements within In2O3 films [, ]. * UV-Vis spectroscopy: Measures optical properties like transmittance and band gap, which are influenced by factors like doping and oxygen vacancies [, , ].* Photoluminescence spectroscopy (PL): Investigates the optical properties and electronic structure of In2O3 nanostructures by analyzing their light emission properties [].
Q3: What factors influence the stability of this compound?
A3: The stability of In2O3 can be affected by:* Temperature: High temperatures can induce phase transitions and alter the material's properties [, , ].* Atmosphere: Exposure to reducing atmospheres can lead to partial reduction and changes in electrical properties [, ].* Doping: The type and concentration of dopants can influence crystal growth, electrical conductivity, and stability [, , , , ].
Q4: Is this compound compatible with flexible substrates for applications like flexible electronics?
A4: Yes, In2O3 can be deposited onto flexible substrates like polyimide (Kapton®) for use in flexible gas sensors []. This compatibility enables the development of flexible and even wearable electronic devices.
Q5: What catalytic applications utilize this compound?
A5: this compound has shown promising catalytic activity in:* CO2 reduction: In2O3 nanoparticles, particularly those with defects, can catalyze the conversion of CO2 to useful products like CO and formate [, , ]. * Water splitting: In2O3 can be used in a reduction-oxidation cycle to decompose water into hydrogen and oxygen, with the process being enhanced by the presence of specific carriers like ZrO2 and TiO2 [].
Q6: How do defects in this compound influence its catalytic activity?
A6: Defects, such as oxygen vacancies and hydroxyl groups, play a crucial role in In2O3's catalytic activity. For instance, in CO2 reduction, these defects can act as active sites, facilitating CO2 adsorption and subsequent reactions [, ]. Theoretical studies using Density Functional Theory (DFT) provide valuable insights into the mechanisms by which these defects enhance catalytic performance [].
Q7: How is computational chemistry used to study this compound?
A7: Computational methods, especially DFT, are vital for understanding and predicting In2O3's properties and behavior:* Electronic structure calculations: DFT helps determine band structures, density of states, and other electronic properties, providing insights into conductivity and optical behavior [, ].* Defect modeling: DFT can model the formation energy and electronic properties of defects in In2O3, helping to explain their influence on catalytic activity [].* Surface interactions: Simulating the interaction of gas molecules like CO2 and H2O with In2O3 surfaces is crucial for understanding catalytic mechanisms [].
Q8: How does the doping of this compound affect its electrical properties?
A8: Doping significantly influences the electrical conductivity of In2O3:* Tin doping (ITO): Widely used in transparent conductors, tin acts as a donor, increasing carrier concentration and enhancing conductivity [, , ].* Zirconium doping: Enhances crystallinity and reduces oxygen vacancies, influencing the electrical properties of solution-processed In2O3 thin films [].* Alkaline-earth metal doping: The type of alkaline-earth metal dopant (Mg, Ca, Sr) influences the dehydroxylation energy and ultimately the TFT performance of this compound [].
Q9: How can the stability of this compound nanoparticles be improved for specific applications?
A9: Strategies for enhancing the stability of In2O3 nanoparticles include:* Surface modifications: Applying protective coatings or ligands can prevent aggregation and enhance stability in various media.* Controlled synthesis: Optimizing synthesis parameters like temperature, pH, and precursor concentration can influence particle size, morphology, and stability.* Dispersion in suitable media: Selecting appropriate solvents or dispersing agents can prevent agglomeration and maintain nanoparticle stability.
Q10: What are the environmental concerns associated with this compound?
A10: Although In2O3 itself is considered relatively benign, the mining and processing of indium can have environmental impacts. These include:* Resource depletion: Indium is a relatively scarce element.* Habitat destruction: Mining activities can disrupt ecosystems.* Chemical pollution: The extraction and purification of indium involve the use of chemicals that require proper handling and disposal.
Q11: Are there regulations regarding the use and disposal of this compound?
A11: While specific regulations targeting In2O3 might vary by region, general guidelines on the handling and disposal of electronic waste (e-waste) often apply. These regulations aim to minimize the environmental impact of potentially hazardous materials found in electronics.
Q12: Are there alternative materials to this compound for transparent conductor applications?
A12: Yes, research is ongoing to find In2O3 substitutes due to factors like indium's cost and scarcity. Some alternatives include:* Aluminum-doped zinc oxide (AZO): A more abundant and less expensive option, but generally with lower conductivity than ITO [, ].* Graphene: Possessing excellent conductivity and transparency, but large-scale production of high-quality graphene remains challenging.* Carbon nanotubes: Exhibiting high conductivity and transparency, but their integration into devices can be complex.
Disclaimer and Information on In-Vitro Research Products
Please be aware that all articles and product information presented on BenchChem are intended solely for informational purposes. The products available for purchase on BenchChem are specifically designed for in-vitro studies, which are conducted outside of living organisms. In-vitro studies, derived from the Latin term "in glass," involve experiments performed in controlled laboratory settings using cells or tissues. It is important to note that these products are not categorized as medicines or drugs, and they have not received approval from the FDA for the prevention, treatment, or cure of any medical condition, ailment, or disease. We must emphasize that any form of bodily introduction of these products into humans or animals is strictly prohibited by law. It is essential to adhere to these guidelines to ensure compliance with legal and ethical standards in research and experimentation.