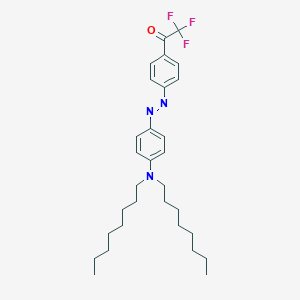
4-(Dioctylamino)-4'-(trifluoroacetyl)azobenzene
Overview
Description
4-(Dioctylamino)-4’-(trifluoroacetyl)azobenzene is an organic compound that belongs to the class of azobenzenes. Azobenzenes are characterized by the presence of a nitrogen-nitrogen double bond (N=N) connecting two aromatic rings. This particular compound features a dioctylamino group and a trifluoroacetyl group, which may impart unique chemical and physical properties.
Preparation Methods
Synthetic Routes and Reaction Conditions
The synthesis of 4-(Dioctylamino)-4’-(trifluoroacetyl)azobenzene typically involves the following steps:
Diazotization: Aniline derivatives are diazotized using nitrous acid to form diazonium salts.
Coupling Reaction: The diazonium salt is then coupled with a suitable aromatic compound to form the azobenzene structure.
Functional Group Modification: Introduction of the dioctylamino and trifluoroacetyl groups through nucleophilic substitution or other suitable reactions.
Industrial Production Methods
Industrial production methods may involve large-scale diazotization and coupling reactions, followed by purification processes such as recrystallization or chromatography to obtain the desired compound in high purity.
Chemical Reactions Analysis
Types of Reactions
4-(Dioctylamino)-4’-(trifluoroacetyl)azobenzene can undergo various chemical reactions, including:
Oxidation: The compound can be oxidized to form different products depending on the reaction conditions.
Reduction: Reduction of the azo group (N=N) can lead to the formation of corresponding amines.
Substitution: The aromatic rings can undergo electrophilic or nucleophilic substitution reactions.
Common Reagents and Conditions
Oxidation: Common oxidizing agents include potassium permanganate (KMnO₄) and chromium trioxide (CrO₃).
Reduction: Reducing agents such as sodium borohydride (NaBH₄) or hydrogen gas (H₂) in the presence of a catalyst can be used.
Substitution: Reagents like halogens (Cl₂, Br₂) or nucleophiles (OH⁻, NH₂⁻) can be employed under appropriate conditions.
Major Products
The major products formed from these reactions depend on the specific reagents and conditions used. For example, reduction of the azo group typically yields amines, while substitution reactions can introduce various functional groups onto the aromatic rings.
Scientific Research Applications
Chemistry: Used as a reagent or intermediate in organic synthesis.
Biology: May be used in studies involving photoresponsive materials due to the azobenzene moiety.
Industry: Utilized in the development of dyes, pigments, and other materials with specific optical properties.
Mechanism of Action
The mechanism of action of 4-(Dioctylamino)-4’-(trifluoroacetyl)azobenzene involves its interaction with molecular targets through various pathways:
Photoisomerization: The azobenzene group can undergo reversible photoisomerization between trans and cis forms upon exposure to light, affecting its physical and chemical properties.
Binding Interactions: The compound may interact with specific proteins, enzymes, or receptors, influencing biological processes.
Comparison with Similar Compounds
Similar Compounds
Azobenzene: The parent compound with a simple N=N linkage between two benzene rings.
4-(Dimethylamino)azobenzene: Similar structure with a dimethylamino group instead of dioctylamino.
4-(Trifluoroacetyl)azobenzene: Similar structure with only the trifluoroacetyl group.
Uniqueness
4-(Dioctylamino)-4’-(trifluoroacetyl)azobenzene is unique due to the presence of both dioctylamino and trifluoroacetyl groups, which may impart distinct chemical reactivity and physical properties compared to other azobenzene derivatives.
Properties
IUPAC Name |
1-[4-[[4-(dioctylamino)phenyl]diazenyl]phenyl]-2,2,2-trifluoroethanone | |
---|---|---|
Source | PubChem | |
URL | https://pubchem.ncbi.nlm.nih.gov | |
Description | Data deposited in or computed by PubChem | |
InChI |
InChI=1S/C30H42F3N3O/c1-3-5-7-9-11-13-23-36(24-14-12-10-8-6-4-2)28-21-19-27(20-22-28)35-34-26-17-15-25(16-18-26)29(37)30(31,32)33/h15-22H,3-14,23-24H2,1-2H3 | |
Source | PubChem | |
URL | https://pubchem.ncbi.nlm.nih.gov | |
Description | Data deposited in or computed by PubChem | |
InChI Key |
LSDDSJZBINXBKD-UHFFFAOYSA-N | |
Source | PubChem | |
URL | https://pubchem.ncbi.nlm.nih.gov | |
Description | Data deposited in or computed by PubChem | |
Canonical SMILES |
CCCCCCCCN(CCCCCCCC)C1=CC=C(C=C1)N=NC2=CC=C(C=C2)C(=O)C(F)(F)F | |
Source | PubChem | |
URL | https://pubchem.ncbi.nlm.nih.gov | |
Description | Data deposited in or computed by PubChem | |
Molecular Formula |
C30H42F3N3O | |
Source | PubChem | |
URL | https://pubchem.ncbi.nlm.nih.gov | |
Description | Data deposited in or computed by PubChem | |
DSSTOX Substance ID |
DTXSID70431382 | |
Record name | Chromoionophore XV | |
Source | EPA DSSTox | |
URL | https://comptox.epa.gov/dashboard/DTXSID70431382 | |
Description | DSSTox provides a high quality public chemistry resource for supporting improved predictive toxicology. | |
Molecular Weight |
517.7 g/mol | |
Source | PubChem | |
URL | https://pubchem.ncbi.nlm.nih.gov | |
Description | Data deposited in or computed by PubChem | |
CAS No. |
193154-07-3 | |
Record name | Chromoionophore XV | |
Source | EPA DSSTox | |
URL | https://comptox.epa.gov/dashboard/DTXSID70431382 | |
Description | DSSTox provides a high quality public chemistry resource for supporting improved predictive toxicology. | |
Retrosynthesis Analysis
AI-Powered Synthesis Planning: Our tool employs the Template_relevance Pistachio, Template_relevance Bkms_metabolic, Template_relevance Pistachio_ringbreaker, Template_relevance Reaxys, Template_relevance Reaxys_biocatalysis model, leveraging a vast database of chemical reactions to predict feasible synthetic routes.
One-Step Synthesis Focus: Specifically designed for one-step synthesis, it provides concise and direct routes for your target compounds, streamlining the synthesis process.
Accurate Predictions: Utilizing the extensive PISTACHIO, BKMS_METABOLIC, PISTACHIO_RINGBREAKER, REAXYS, REAXYS_BIOCATALYSIS database, our tool offers high-accuracy predictions, reflecting the latest in chemical research and data.
Strategy Settings
Precursor scoring | Relevance Heuristic |
---|---|
Min. plausibility | 0.01 |
Model | Template_relevance |
Template Set | Pistachio/Bkms_metabolic/Pistachio_ringbreaker/Reaxys/Reaxys_biocatalysis |
Top-N result to add to graph | 6 |
Feasible Synthetic Routes
Q1: How does 4-(Dioctylamino)-4'-(trifluoroacetyl)azobenzene interact with amines and what are the observable effects?
A1: this compound demonstrates a distinct optical response upon interaction with various amines. [] This response manifests as a detectable change in its optical properties, making it suitable for sensing applications. For instance, exposure to ammonia, tetramethylammonium hydroxide, ethylamine, cadaverine, and putrescine—compounds indicative of protein decomposition—results in observable optical changes. [] This interaction forms the basis for its use in developing optical sensors for amine detection.
Q2: What are the potential applications of this compound in food packaging?
A2: Research suggests that thin films of this compound, deposited via inkjet printing, could be integrated into food packaging for ground meat and salmon. [] As these foods degrade, they release biogenic amines. The dye's sensitivity to these amines would enable the detection of food spoilage, potentially improving safety and quality control in the food industry.
Q3: How does incorporating this compound into a carbon nanotube-Nafion® composite enhance its sensing capabilities?
A3: Combining this compound with carbon nanotubes and Nafion® creates a synergistic effect for electrochemical amine sensing. [] The carbon nanotubes provide a large surface area for amine adsorption, enhancing the sensor's sensitivity. Additionally, their inherent electrical conductivity facilitates efficient charge transfer within the sensor, enabling electrochemical detection of the amine-dye interaction. This composite demonstrates the potential for developing sensitive and selective electrochemical amine sensors.
Disclaimer and Information on In-Vitro Research Products
Please be aware that all articles and product information presented on BenchChem are intended solely for informational purposes. The products available for purchase on BenchChem are specifically designed for in-vitro studies, which are conducted outside of living organisms. In-vitro studies, derived from the Latin term "in glass," involve experiments performed in controlled laboratory settings using cells or tissues. It is important to note that these products are not categorized as medicines or drugs, and they have not received approval from the FDA for the prevention, treatment, or cure of any medical condition, ailment, or disease. We must emphasize that any form of bodily introduction of these products into humans or animals is strictly prohibited by law. It is essential to adhere to these guidelines to ensure compliance with legal and ethical standards in research and experimentation.