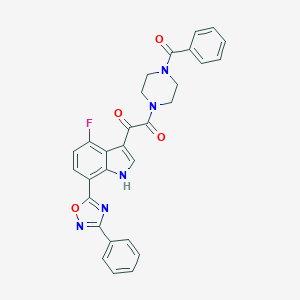
Myrislignan
Overview
Description
Myrislignan is a bioactive 8-O-4’-neolignan compound found in nutmeg, which is derived from the seeds of Myristica fragrans. This compound is recognized for its various pharmacological activities, including anti-inflammatory, antioxidant, and anti-cancer properties . This compound is an official Traditional Chinese Medicine used for the treatment of gastrointestinal diseases in China .
Mechanism of Action
Target of Action
Myrislignan, a natural compound isolated from Myristica fragrans Houtt, has been found to target several key proteins and pathways in cells. It primarily targets the extracellular signal-regulated kinase (ERK) and NF-kB signalling pathway . ERK is a key player in the regulation of cell functions such as proliferation and differentiation . NF-kB is a protein complex that controls the transcription of DNA and plays a key role in regulating the immune response to infection .
Mode of Action
This compound interacts with its targets, leading to significant changes in cellular processes. It inhibits the phosphorylation of ERK by suppressing mitochondrial function . This leads to the downregulation of the nuclear factor of activated T cells 1 (NFATc1) signaling . Furthermore, it attenuates inflammation in murine macrophage cells through inhibition of NF-kB signalling pathway activation .
Biochemical Pathways
This compound affects several biochemical pathways. It impacts the oxidation-reduction process in Toxoplasma gondii, leading to an upregulation of ROS activity and disruption of the oxidant-antioxidant homeostasis of tachyzoites . It also affects the mitogen-activated protein kinase (MAPK) and nuclear factor-κB pathways .
Pharmacokinetics
The pharmacokinetics of this compound have been studied in mice. After a single oral administration of 200 mg/kg or intraperitoneal administration of 50 mg/kg, the bioavailability (F) of orally administered this compound was found to be only 1.97% of the bioavailability of intraperitoneally administered this compound . This suggests that the route of administration significantly impacts the bioavailability of this compound.
Result of Action
The action of this compound results in significant molecular and cellular effects. It induces redox imbalance and activates autophagy in Toxoplasma gondii . It also inhibits osteoclast differentiation and associated bone resorption by significantly decreasing osteoclastic gene expression . In A549 cells, this compound has been shown to induce apoptosis and cell cycle arrest .
Action Environment
The action of this compound can be influenced by environmental factors. For instance, the internal redox environment of a cell can impact the effectiveness of this compound. In an environment of imbalanced internal redox system caused by this compound, there is an upregulation of ROS activity . This suggests that the cellular environment plays a crucial role in the action and efficacy of this compound.
Biochemical Analysis
Biochemical Properties
Myrislignan interacts with various enzymes and proteins. It has been found that hydroxylation and demethylation are the major metabolic pathways for this compound metabolism in vitro and in vivo, respectively. Recombinant cytochrome P450s (CYPs) screening revealed that CYP3A4 and CYP3A5 play a crucial role in the metabolism of this compound .
Cellular Effects
This compound has been shown to have significant effects on various types of cells and cellular processes. It has been found to induce redox imbalance and activate autophagy in Toxoplasma gondii . In addition, it has been shown to inhibit the proliferation of A549 cells in a dose- and time-dependent manner .
Molecular Mechanism
This compound exerts its effects at the molecular level through various mechanisms. It inhibits the phosphorylation of extracellular signal-regulated kinase (ERK) by suppressing mitochondrial function, thereby downregulating the nuclear factor of activated T cells 1 (NFATc1) signaling . It also induces apoptosis and cell cycle arrest in A549 cells through the activation of mitogen-activated protein kinase and the inhibition of epidermal growth factor receptor signal pathway .
Temporal Effects in Laboratory Settings
The effects of this compound change over time in laboratory settings. A study has shown that this compound levels were determined in mouse plasma following a single oral administration of 200 mg/kg or intraperitoneal administration of 50 mg/kg this compound .
Dosage Effects in Animal Models
The effects of this compound vary with different dosages in animal models. For instance, a study has shown that this compound levels were determined in mouse plasma following a single oral administration of 200 mg/kg or intraperitoneal administration of 50 mg/kg this compound .
Metabolic Pathways
This compound is involved in various metabolic pathways. It has been found that hydroxylation and demethylation are the major metabolic pathways for this compound metabolism in vitro and in vivo, respectively .
Transport and Distribution
This compound is transported and distributed within cells and tissues. A study has shown that this compound levels were determined in rat tissues by a high-performance liquid chromatography–ultraviolet detection (HPLC–UV) method .
Preparation Methods
Synthetic Routes and Reaction Conditions: Myrislignan can be synthesized through the dimerization of monolignols to form a dibenzylbutane skeleton. The synthetic route involves the coupling of two phenylpropanoid units via an 8-O-4’ bond . The reaction conditions typically include the use of catalysts and specific temperature and pressure settings to facilitate the dimerization process.
Industrial Production Methods: Industrial production of this compound involves the extraction of the compound from Myristica fragrans seeds. The extraction process includes solvent extraction, followed by purification using techniques such as ultra-high performance liquid chromatography coupled with mass spectrometry (UHPLC-MS) . This method ensures the isolation of this compound in its pure form for further applications.
Chemical Reactions Analysis
Types of Reactions: Myrislignan undergoes various chemical reactions, including hydroxylation, demethylation, and oxidation . These reactions are crucial for the metabolism and disposition of this compound in biological systems.
Common Reagents and Conditions:
Hydroxylation: Typically involves the use of hydroxylating agents under controlled temperature and pH conditions.
Demethylation: Requires demethylating agents such as boron tribromide or aluminum chloride.
Oxidation: Involves the use of oxidizing agents like potassium permanganate or hydrogen peroxide.
Major Products Formed: The major products formed from these reactions include hydroxylated and demethylated derivatives of this compound, which exhibit enhanced pharmacological activities .
Scientific Research Applications
Myrislignan has a wide range of scientific research applications:
Chemistry: Used as a model compound for studying the dimerization of phenylpropanoids and the formation of neolignans.
Biology: Investigated for its role in modulating cellular pathways and gene expression.
Medicine: Explored for its potential therapeutic effects in treating cancer, inflammation, and osteoporosis
Industry: Utilized in the development of nutraceuticals and functional foods due to its antioxidant properties.
Comparison with Similar Compounds
Myrislignan is unique compared to other lignans due to its specific 8-O-4’ bond and its distribution in nutmeg. Similar compounds include:
Podophyllotoxin: Known for its anti-cancer properties but differs in its molecular structure and source.
Sesamin: Another lignan with antioxidant and anti-inflammatory properties, found in sesame seeds.
Matairesinol: Exhibits similar pharmacological activities but has a different structural configuration
This compound’s distinct structure and diverse pharmacological activities make it a valuable compound for various scientific and industrial applications.
Properties
IUPAC Name |
4-[(1R,2S)-2-(2,6-dimethoxy-4-prop-2-enylphenoxy)-1-hydroxypropyl]-2-methoxyphenol | |
---|---|---|
Source | PubChem | |
URL | https://pubchem.ncbi.nlm.nih.gov | |
Description | Data deposited in or computed by PubChem | |
InChI |
InChI=1S/C21H26O6/c1-6-7-14-10-18(25-4)21(19(11-14)26-5)27-13(2)20(23)15-8-9-16(22)17(12-15)24-3/h6,8-13,20,22-23H,1,7H2,2-5H3/t13-,20-/m0/s1 | |
Source | PubChem | |
URL | https://pubchem.ncbi.nlm.nih.gov | |
Description | Data deposited in or computed by PubChem | |
InChI Key |
ULZFTGWWPHYLGI-RBZFPXEDSA-N | |
Source | PubChem | |
URL | https://pubchem.ncbi.nlm.nih.gov | |
Description | Data deposited in or computed by PubChem | |
Canonical SMILES |
CC(C(C1=CC(=C(C=C1)O)OC)O)OC2=C(C=C(C=C2OC)CC=C)OC | |
Source | PubChem | |
URL | https://pubchem.ncbi.nlm.nih.gov | |
Description | Data deposited in or computed by PubChem | |
Isomeric SMILES |
C[C@@H]([C@@H](C1=CC(=C(C=C1)O)OC)O)OC2=C(C=C(C=C2OC)CC=C)OC | |
Source | PubChem | |
URL | https://pubchem.ncbi.nlm.nih.gov | |
Description | Data deposited in or computed by PubChem | |
Molecular Formula |
C21H26O6 | |
Source | PubChem | |
URL | https://pubchem.ncbi.nlm.nih.gov | |
Description | Data deposited in or computed by PubChem | |
DSSTOX Substance ID |
DTXSID30333494 | |
Record name | (-)-(1R,2S)-Myrislignan | |
Source | EPA DSSTox | |
URL | https://comptox.epa.gov/dashboard/DTXSID30333494 | |
Description | DSSTox provides a high quality public chemistry resource for supporting improved predictive toxicology. | |
Molecular Weight |
374.4 g/mol | |
Source | PubChem | |
URL | https://pubchem.ncbi.nlm.nih.gov | |
Description | Data deposited in or computed by PubChem | |
CAS No. |
171485-39-5 | |
Record name | (-)-(1R,2S)-Myrislignan | |
Source | EPA DSSTox | |
URL | https://comptox.epa.gov/dashboard/DTXSID30333494 | |
Description | DSSTox provides a high quality public chemistry resource for supporting improved predictive toxicology. | |
Retrosynthesis Analysis
AI-Powered Synthesis Planning: Our tool employs the Template_relevance Pistachio, Template_relevance Bkms_metabolic, Template_relevance Pistachio_ringbreaker, Template_relevance Reaxys, Template_relevance Reaxys_biocatalysis model, leveraging a vast database of chemical reactions to predict feasible synthetic routes.
One-Step Synthesis Focus: Specifically designed for one-step synthesis, it provides concise and direct routes for your target compounds, streamlining the synthesis process.
Accurate Predictions: Utilizing the extensive PISTACHIO, BKMS_METABOLIC, PISTACHIO_RINGBREAKER, REAXYS, REAXYS_BIOCATALYSIS database, our tool offers high-accuracy predictions, reflecting the latest in chemical research and data.
Strategy Settings
Precursor scoring | Relevance Heuristic |
---|---|
Min. plausibility | 0.01 |
Model | Template_relevance |
Template Set | Pistachio/Bkms_metabolic/Pistachio_ringbreaker/Reaxys/Reaxys_biocatalysis |
Top-N result to add to graph | 6 |
Feasible Synthetic Routes
Disclaimer and Information on In-Vitro Research Products
Please be aware that all articles and product information presented on BenchChem are intended solely for informational purposes. The products available for purchase on BenchChem are specifically designed for in-vitro studies, which are conducted outside of living organisms. In-vitro studies, derived from the Latin term "in glass," involve experiments performed in controlled laboratory settings using cells or tissues. It is important to note that these products are not categorized as medicines or drugs, and they have not received approval from the FDA for the prevention, treatment, or cure of any medical condition, ailment, or disease. We must emphasize that any form of bodily introduction of these products into humans or animals is strictly prohibited by law. It is essential to adhere to these guidelines to ensure compliance with legal and ethical standards in research and experimentation.