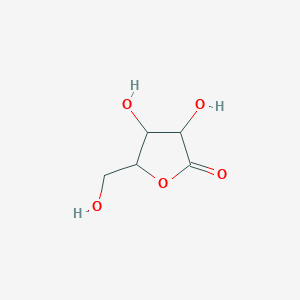
3,4-Dihydroxy-5-(hydroxymethyl)oxolan-2-one
Overview
Description
D-Ribonolactone: is a carbohydrate derivative belonging to the aldonolactone family. It is a five-membered lactone (γ-lactone) with the molecular formula C5H8O5 and a molecular weight of 148.11 g/mol . This compound is a versatile chiral pool used in the synthesis of various natural products and biologically relevant scaffolds .
Preparation Methods
Synthetic Routes and Reaction Conditions: D-Ribonolactone can be synthesized from D-ribose through selective anomeric oxidation using bromine. The process involves dissolving D-ribose in water with sodium bicarbonate, followed by the addition of bromine at a controlled temperature to prevent excessive heat . The reaction mixture is then treated with sodium bisulfite to remove any residual bromine, and the product is crystallized from ethanol .
Industrial Production Methods: Industrial production of D-Ribonolactone often involves the use of continuous flow microchannel reactors, which offer advantages such as shorter reaction times and higher purity of the final product .
Chemical Reactions Analysis
Types of Reactions: D-Ribonolactone undergoes various chemical reactions, including:
Oxidation: It can be oxidized to form D-ribonate.
Reduction: Reduction reactions can convert it into different sugar alcohols.
Common Reagents and Conditions:
Oxidation: Bromine in aqueous solution, followed by sodium bisulfite.
Reduction: Catalytic hydrogenation using metal catalysts.
Substitution: Nucleophiles such as amines or thiols under basic conditions.
Major Products:
D-Ribonate: Formed through oxidation.
C-Nucleosides: Formed through nucleophilic substitution reactions.
Scientific Research Applications
Biological Applications
3,4-Dihydroxy-5-(hydroxymethyl)oxolan-2-one has been investigated for its potential roles in various biological processes:
- Enzyme Mechanisms : It serves as a probe for studying enzyme mechanisms, particularly in glycosylation reactions. Its structural similarity to natural substrates allows it to mimic interactions with enzymes involved in carbohydrate metabolism.
- Antiviral and Anticancer Properties : Preliminary studies suggest that the compound may exhibit antiviral and anticancer activities. It is being explored as a drug candidate due to its ability to inhibit specific enzymes that are crucial for viral replication and cancer cell proliferation.
Chemical Synthesis
The compound acts as a versatile building block in organic synthesis:
- Synthesis of Complex Molecules : It is utilized in the synthesis of more complex organic molecules, including nucleoside analogs and other glycosylamines. The presence of hydroxyl groups facilitates various chemical reactions such as oxidation and reduction.
Pharmaceutical Development
In the pharmaceutical industry, this compound is being studied for:
- Drug Formulation : Its properties make it suitable for developing new therapeutic agents targeting metabolic diseases and infections. The compound's ability to interact with biological macromolecules enhances its potential as a lead compound in drug design.
Case Study 1: Antiviral Activity
A study demonstrated that derivatives of this compound exhibited significant antiviral activity against specific viruses by inhibiting their replication processes. The mechanism involved competitive inhibition of viral polymerases, making it a promising candidate for further development in antiviral therapies.
Case Study 2: Enzyme Inhibition
Research focused on the use of this compound as an inhibitor of glycosyltransferases showed that it effectively reduced enzyme activity in vitro. This inhibition suggests potential applications in treating diseases related to abnormal glycosylation patterns.
Comparative Data Table
Application Area | Description | Key Findings |
---|---|---|
Biological Research | Enzyme mechanisms and biological interactions | Effective probe for studying glycosylation enzymes |
Pharmaceutical Development | Drug candidate for antiviral and anticancer therapies | Exhibits significant activity against viral replication |
Chemical Synthesis | Building block for complex organic molecules | Facilitates synthesis of nucleoside analogs |
Mechanism of Action
D-Ribonolactone acts as an inhibitor of β-galactosidase in Escherichia coli, with a Ki of 26 mM . The inhibition mechanism involves binding to the active site of the enzyme, preventing the hydrolysis of β-galactosides. This inhibition can be utilized in various biochemical assays and research applications.
Comparison with Similar Compounds
D-Gluconolactone: Another aldonolactone with a six-membered ring (δ-lactone) that is commonly used in food and pharmaceutical industries.
L-Ribonolactone: The L-isomer of D-Ribonolactone, which has similar chemical properties but different biological activities.
Uniqueness: D-Ribonolactone is unique due to its five-membered ring structure, which provides distinct reactivity and stability compared to six-membered lactones like D-Gluconolactone . Its ability to serve as a chiral pool for the synthesis of various natural products and its role as a precursor for C-nucleosides highlight its importance in both synthetic chemistry and medicinal research .
Biological Activity
3,4-Dihydroxy-5-(hydroxymethyl)oxolan-2-one, also known as a derivative of 1-beta-D-arabinofuranosyl-5-iodouracil, is a compound of significant interest in medicinal chemistry due to its potential therapeutic applications. This article explores its biological activity, mechanisms of action, and potential applications in various fields.
Chemical Structure and Properties
The molecular formula of this compound is , and it possesses a unique stereochemistry that influences its biological activity. The compound features multiple hydroxyl groups that enhance its interaction with biological macromolecules.
The biological activity of this compound is primarily attributed to its ability to interact with various enzymes and receptors. Key mechanisms include:
- Inhibition of DNA Synthesis : It has been shown to inhibit DNA replication by interfering with the activity of DNA polymerases.
- Induction of Apoptosis : The compound can trigger programmed cell death in cancer cells, making it a candidate for anticancer therapies.
- Modulation of Signaling Pathways : It influences intracellular signaling pathways that regulate cell growth and differentiation.
Biological Activities
Research has documented several biological activities associated with this compound:
- Antiviral Activity : Studies indicate that this compound exhibits antiviral properties against various viruses, including poxviruses .
- Anticancer Potential : Its ability to induce apoptosis in malignant cells positions it as a promising agent in cancer treatment.
- Neuroprotective Effects : Emerging evidence suggests potential neuroprotective properties, which may be beneficial in treating neurodegenerative diseases.
Case Studies
- Antiviral Efficacy : A study examining the interaction between this compound and the cowpox virus demonstrated significant inhibition of viral replication. The compound formed hydrogen bonds with critical viral proteins, disrupting their function .
- Cancer Cell Studies : In vitro studies on various cancer cell lines showed that treatment with this compound led to a marked decrease in cell viability and increased apoptosis rates. The mechanism involved the activation of caspase pathways.
- Neuroprotection : Research involving animal models indicated that administration of this compound resulted in reduced neuronal damage following ischemic events. The proposed mechanism involves antioxidant activity and modulation of inflammatory responses.
Comparative Analysis
The following table summarizes the biological activities and mechanisms of action for this compound compared to similar compounds:
Compound Name | Biological Activity | Mechanism of Action |
---|---|---|
This compound | Antiviral, Anticancer | Inhibition of DNA synthesis, Apoptosis |
1-beta-D-arabinofuranosyl-5-iodouracil | Antiviral | Nucleoside analog inhibiting viral replication |
Quercetin | Antioxidant | Scavenging free radicals |
Curcumin | Anti-inflammatory | Modulation of inflammatory pathways |
Properties
IUPAC Name |
3,4-dihydroxy-5-(hydroxymethyl)oxolan-2-one | |
---|---|---|
Source | PubChem | |
URL | https://pubchem.ncbi.nlm.nih.gov | |
Description | Data deposited in or computed by PubChem | |
InChI |
InChI=1S/C5H8O5/c6-1-2-3(7)4(8)5(9)10-2/h2-4,6-8H,1H2 | |
Source | PubChem | |
URL | https://pubchem.ncbi.nlm.nih.gov | |
Description | Data deposited in or computed by PubChem | |
InChI Key |
CUOKHACJLGPRHD-UHFFFAOYSA-N | |
Source | PubChem | |
URL | https://pubchem.ncbi.nlm.nih.gov | |
Description | Data deposited in or computed by PubChem | |
Canonical SMILES |
C(C1C(C(C(=O)O1)O)O)O | |
Source | PubChem | |
URL | https://pubchem.ncbi.nlm.nih.gov | |
Description | Data deposited in or computed by PubChem | |
Molecular Formula |
C5H8O5 | |
Source | PubChem | |
URL | https://pubchem.ncbi.nlm.nih.gov | |
Description | Data deposited in or computed by PubChem | |
DSSTOX Substance ID |
DTXSID50863521 | |
Record name | 3,4-Dihydroxy-5-(hydroxymethyl)oxolan-2-one (non-preferred name) | |
Source | EPA DSSTox | |
URL | https://comptox.epa.gov/dashboard/DTXSID50863521 | |
Description | DSSTox provides a high quality public chemistry resource for supporting improved predictive toxicology. | |
Molecular Weight |
148.11 g/mol | |
Source | PubChem | |
URL | https://pubchem.ncbi.nlm.nih.gov | |
Description | Data deposited in or computed by PubChem | |
CAS No. |
5336-08-3 | |
Record name | D-Ribonolactone | |
Source | DTP/NCI | |
URL | https://dtp.cancer.gov/dtpstandard/servlet/dwindex?searchtype=NSC&outputformat=html&searchlist=1031 | |
Description | The NCI Development Therapeutics Program (DTP) provides services and resources to the academic and private-sector research communities worldwide to facilitate the discovery and development of new cancer therapeutic agents. | |
Explanation | Unless otherwise indicated, all text within NCI products is free of copyright and may be reused without our permission. Credit the National Cancer Institute as the source. | |
Retrosynthesis Analysis
AI-Powered Synthesis Planning: Our tool employs the Template_relevance Pistachio, Template_relevance Bkms_metabolic, Template_relevance Pistachio_ringbreaker, Template_relevance Reaxys, Template_relevance Reaxys_biocatalysis model, leveraging a vast database of chemical reactions to predict feasible synthetic routes.
One-Step Synthesis Focus: Specifically designed for one-step synthesis, it provides concise and direct routes for your target compounds, streamlining the synthesis process.
Accurate Predictions: Utilizing the extensive PISTACHIO, BKMS_METABOLIC, PISTACHIO_RINGBREAKER, REAXYS, REAXYS_BIOCATALYSIS database, our tool offers high-accuracy predictions, reflecting the latest in chemical research and data.
Strategy Settings
Precursor scoring | Relevance Heuristic |
---|---|
Min. plausibility | 0.01 |
Model | Template_relevance |
Template Set | Pistachio/Bkms_metabolic/Pistachio_ringbreaker/Reaxys/Reaxys_biocatalysis |
Top-N result to add to graph | 6 |
Feasible Synthetic Routes
Disclaimer and Information on In-Vitro Research Products
Please be aware that all articles and product information presented on BenchChem are intended solely for informational purposes. The products available for purchase on BenchChem are specifically designed for in-vitro studies, which are conducted outside of living organisms. In-vitro studies, derived from the Latin term "in glass," involve experiments performed in controlled laboratory settings using cells or tissues. It is important to note that these products are not categorized as medicines or drugs, and they have not received approval from the FDA for the prevention, treatment, or cure of any medical condition, ailment, or disease. We must emphasize that any form of bodily introduction of these products into humans or animals is strictly prohibited by law. It is essential to adhere to these guidelines to ensure compliance with legal and ethical standards in research and experimentation.