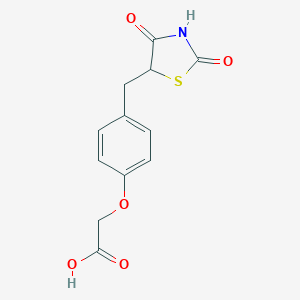
2-(4-((2,4-Dioxothiazolidin-5-YL)methyl)phenoxy)acetic acid
Overview
Description
2-(4-((2,4-Dioxothiazolidin-5-YL)methyl)phenoxy)acetic acid is a synthetic organic compound with the molecular formula C12H11NO5S. It is characterized by the presence of a thiazolidine ring, which is a five-membered ring containing both sulfur and nitrogen atoms.
Preparation Methods
Synthetic Routes and Reaction Conditions
The synthesis of 2-(4-((2,4-Dioxothiazolidin-5-YL)methyl)phenoxy)acetic acid typically involves the reaction of thiourea with maleic anhydride in the presence of hydrochloric acid. This one-pot method is efficient and yields the desired product with high purity . The reaction conditions include maintaining an inert atmosphere and a temperature range of 2-8°C .
Industrial Production Methods
Industrial production of this compound follows similar synthetic routes but on a larger scale. The process involves the use of automated reactors and precise control of reaction parameters to ensure consistent quality and yield. The compound is then purified using standard techniques such as recrystallization or chromatography .
Chemical Reactions Analysis
Types of Reactions
2-(4-((2,4-Dioxothiazolidin-5-YL)methyl)phenoxy)acetic acid undergoes various chemical reactions, including:
Oxidation: The compound can be oxidized to form sulfoxides or sulfones.
Reduction: Reduction reactions can convert the carbonyl groups to alcohols.
Substitution: The phenoxy group can undergo nucleophilic substitution reactions.
Common Reagents and Conditions
Oxidation: Common oxidizing agents include hydrogen peroxide and potassium permanganate.
Reduction: Reducing agents such as sodium borohydride or lithium aluminum hydride are used.
Substitution: Nucleophiles like amines or thiols can be used for substitution reactions.
Major Products Formed
The major products formed from these reactions include sulfoxides, sulfones, alcohols, and substituted phenoxy derivatives .
Scientific Research Applications
2-(4-((2,4-Dioxothiazolidin-5-YL)methyl)phenoxy)acetic acid has several scientific research applications:
Chemistry: It is used as a building block for the synthesis of more complex molecules.
Biology: The compound has been studied for its potential antioxidant properties.
Medicine: Research has shown its potential antimicrobial activity, making it a candidate for drug development.
Industry: It is used in the development of new materials with specific properties.
Mechanism of Action
The mechanism of action of 2-(4-((2,4-Dioxothiazolidin-5-YL)methyl)phenoxy)acetic acid involves its interaction with biological molecules. The thiazolidine ring can form hydrogen bonds with proteins and DNA, leading to changes in their structure and function. This interaction can result in antioxidant and antimicrobial effects .
Comparison with Similar Compounds
Similar Compounds
2,4-Dioxothiazolidine-5-acetic acid: A similar compound with a thiazolidine ring but different substituents.
Thiazolidinedione derivatives: Compounds with similar core structures but varying functional groups.
Uniqueness
2-(4-((2,4-Dioxothiazolidin-5-YL)methyl)phenoxy)acetic acid is unique due to its specific combination of a thiazolidine ring and a phenoxyacetic acid moiety. This structure imparts distinct chemical and biological properties, making it valuable for various applications .
Properties
IUPAC Name |
2-[4-[(2,4-dioxo-1,3-thiazolidin-5-yl)methyl]phenoxy]acetic acid | |
---|---|---|
Source | PubChem | |
URL | https://pubchem.ncbi.nlm.nih.gov | |
Description | Data deposited in or computed by PubChem | |
InChI |
InChI=1S/C12H11NO5S/c14-10(15)6-18-8-3-1-7(2-4-8)5-9-11(16)13-12(17)19-9/h1-4,9H,5-6H2,(H,14,15)(H,13,16,17) | |
Source | PubChem | |
URL | https://pubchem.ncbi.nlm.nih.gov | |
Description | Data deposited in or computed by PubChem | |
InChI Key |
SXAASESEPRXRTR-UHFFFAOYSA-N | |
Source | PubChem | |
URL | https://pubchem.ncbi.nlm.nih.gov | |
Description | Data deposited in or computed by PubChem | |
Canonical SMILES |
C1=CC(=CC=C1CC2C(=O)NC(=O)S2)OCC(=O)O | |
Source | PubChem | |
URL | https://pubchem.ncbi.nlm.nih.gov | |
Description | Data deposited in or computed by PubChem | |
Molecular Formula |
C12H11NO5S | |
Source | PubChem | |
URL | https://pubchem.ncbi.nlm.nih.gov | |
Description | Data deposited in or computed by PubChem | |
DSSTOX Substance ID |
DTXSID80462405 | |
Record name | 2-(4-((2,4-DIOXOTHIAZOLIDIN-5-YL)METHYL)PHENOXY)ACETIC ACID | |
Source | EPA DSSTox | |
URL | https://comptox.epa.gov/dashboard/DTXSID80462405 | |
Description | DSSTox provides a high quality public chemistry resource for supporting improved predictive toxicology. | |
Molecular Weight |
281.29 g/mol | |
Source | PubChem | |
URL | https://pubchem.ncbi.nlm.nih.gov | |
Description | Data deposited in or computed by PubChem | |
CAS No. |
179087-93-5 | |
Record name | 2-(4-((2,4-DIOXOTHIAZOLIDIN-5-YL)METHYL)PHENOXY)ACETIC ACID | |
Source | EPA DSSTox | |
URL | https://comptox.epa.gov/dashboard/DTXSID80462405 | |
Description | DSSTox provides a high quality public chemistry resource for supporting improved predictive toxicology. | |
Synthesis routes and methods I
Procedure details
Synthesis routes and methods II
Procedure details
Synthesis routes and methods III
Procedure details
Retrosynthesis Analysis
AI-Powered Synthesis Planning: Our tool employs the Template_relevance Pistachio, Template_relevance Bkms_metabolic, Template_relevance Pistachio_ringbreaker, Template_relevance Reaxys, Template_relevance Reaxys_biocatalysis model, leveraging a vast database of chemical reactions to predict feasible synthetic routes.
One-Step Synthesis Focus: Specifically designed for one-step synthesis, it provides concise and direct routes for your target compounds, streamlining the synthesis process.
Accurate Predictions: Utilizing the extensive PISTACHIO, BKMS_METABOLIC, PISTACHIO_RINGBREAKER, REAXYS, REAXYS_BIOCATALYSIS database, our tool offers high-accuracy predictions, reflecting the latest in chemical research and data.
Strategy Settings
Precursor scoring | Relevance Heuristic |
---|---|
Min. plausibility | 0.01 |
Model | Template_relevance |
Template Set | Pistachio/Bkms_metabolic/Pistachio_ringbreaker/Reaxys/Reaxys_biocatalysis |
Top-N result to add to graph | 6 |
Feasible Synthetic Routes
Disclaimer and Information on In-Vitro Research Products
Please be aware that all articles and product information presented on BenchChem are intended solely for informational purposes. The products available for purchase on BenchChem are specifically designed for in-vitro studies, which are conducted outside of living organisms. In-vitro studies, derived from the Latin term "in glass," involve experiments performed in controlled laboratory settings using cells or tissues. It is important to note that these products are not categorized as medicines or drugs, and they have not received approval from the FDA for the prevention, treatment, or cure of any medical condition, ailment, or disease. We must emphasize that any form of bodily introduction of these products into humans or animals is strictly prohibited by law. It is essential to adhere to these guidelines to ensure compliance with legal and ethical standards in research and experimentation.