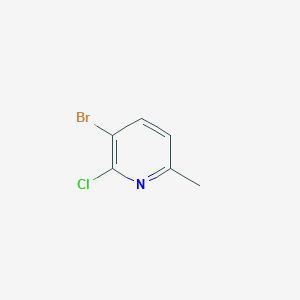
3-Bromo-2-chloro-6-methylpyridine
Overview
Description
3-Bromo-2-chloro-6-methylpyridine: is a halogenated heterocyclic compound with the molecular formula C6H5BrClN and a molecular weight of 206.47 g/mol . It is a derivative of pyridine, characterized by the presence of bromine, chlorine, and a methyl group attached to the pyridine ring. This compound is used in various chemical syntheses and has applications in different fields of scientific research .
Mechanism of Action
Target of Action
It’s known that halogenated pyridines, such as this compound, often interact with various biological targets, including enzymes and receptors, in the respiratory system .
Mode of Action
These interactions can lead to changes in the target’s structure or function, potentially altering biochemical pathways .
Biochemical Pathways
For instance, they may inhibit or activate enzymes, modulate receptor activity, or interfere with cellular processes .
Pharmacokinetics
Its molecular weight (206.47 g/mol) suggests it could potentially cross biological membranes .
Result of Action
Given its potential targets in the respiratory system, it could potentially cause respiratory irritation .
Action Environment
Environmental factors can significantly influence the action, efficacy, and stability of 3-Bromo-2-chloro-6-methylpyridine. Factors such as temperature, pH, and the presence of other molecules can affect its solubility, stability, and interactions with its targets .
Preparation Methods
Synthetic Routes and Reaction Conditions: The synthesis of 3-Bromo-2-chloro-6-methylpyridine typically involves the halogenation of 2-amino-6-methyl-5-bromopyridine. One common method includes the following steps :
Starting Material: 2-amino-6-methyl-5-bromopyridine.
Reagents: Pyridine-HCl, NaNO2, CuCl.
Solvent: Dichloromethane (CH2Cl2).
Reaction Conditions: The mixture is cooled to 0-10°C in an ice-water bath and then allowed to react.
Industrial Production Methods: Industrial production methods for this compound are similar to laboratory synthesis but are scaled up to accommodate larger quantities. The process involves the use of large reactors, precise temperature control, and efficient purification techniques to ensure high yield and purity .
Chemical Reactions Analysis
Types of Reactions: 3-Bromo-2-chloro-6-methylpyridine undergoes various chemical reactions, including:
Substitution Reactions: The bromine or chlorine atoms can be substituted by other nucleophiles.
Oxidation and Reduction Reactions: The compound can be oxidized or reduced under specific conditions.
Common Reagents and Conditions:
Substitution Reactions: Common reagents include sodium hydride (NaH), potassium tert-butoxide (KOtBu), and various nucleophiles.
Oxidation Reactions: Reagents such as potassium permanganate (KMnO4) or chromium trioxide (CrO3) can be used.
Reduction Reactions: Reagents like lithium aluminum hydride (LiAlH4) or sodium borohydride (NaBH4) are commonly employed.
Major Products: The major products formed from these reactions depend on the specific reagents and conditions used. For example, substitution reactions can yield various substituted pyridines, while oxidation and reduction reactions can lead to different oxidized or reduced derivatives .
Scientific Research Applications
Chemistry: 3-Bromo-2-chloro-6-methylpyridine is used as a building block in organic synthesis. It is employed in the preparation of various heterocyclic compounds and as an intermediate in the synthesis of pharmaceuticals and agrochemicals .
Biology and Medicine: In biological research, this compound is used to study the effects of halogenated pyridines on biological systems.
Industry: In the industrial sector, this compound is used in the production of specialty chemicals and as a precursor for the synthesis of more complex molecules .
Comparison with Similar Compounds
- 2-Bromo-6-methylpyridine
- 3-Bromo-6-chloro-2-methylpyridine
- 5-Bromo-2-chloro-4-methylpyridine
- 2-Amino-5-bromopyridine
Comparison: 3-Bromo-2-chloro-6-methylpyridine is unique due to the specific positioning of the bromine, chlorine, and methyl groups on the pyridine ring. This unique structure imparts distinct chemical and physical properties, making it suitable for specific applications in synthesis and research .
Properties
IUPAC Name |
3-bromo-2-chloro-6-methylpyridine | |
---|---|---|
Source | PubChem | |
URL | https://pubchem.ncbi.nlm.nih.gov | |
Description | Data deposited in or computed by PubChem | |
InChI |
InChI=1S/C6H5BrClN/c1-4-2-3-5(7)6(8)9-4/h2-3H,1H3 | |
Source | PubChem | |
URL | https://pubchem.ncbi.nlm.nih.gov | |
Description | Data deposited in or computed by PubChem | |
InChI Key |
JVDQYSIJBRTRMS-UHFFFAOYSA-N | |
Source | PubChem | |
URL | https://pubchem.ncbi.nlm.nih.gov | |
Description | Data deposited in or computed by PubChem | |
Canonical SMILES |
CC1=NC(=C(C=C1)Br)Cl | |
Source | PubChem | |
URL | https://pubchem.ncbi.nlm.nih.gov | |
Description | Data deposited in or computed by PubChem | |
Molecular Formula |
C6H5BrClN | |
Source | PubChem | |
URL | https://pubchem.ncbi.nlm.nih.gov | |
Description | Data deposited in or computed by PubChem | |
DSSTOX Substance ID |
DTXSID20370297 | |
Record name | 3-bromo-2-chloro-6-picoline | |
Source | EPA DSSTox | |
URL | https://comptox.epa.gov/dashboard/DTXSID20370297 | |
Description | DSSTox provides a high quality public chemistry resource for supporting improved predictive toxicology. | |
Molecular Weight |
206.47 g/mol | |
Source | PubChem | |
URL | https://pubchem.ncbi.nlm.nih.gov | |
Description | Data deposited in or computed by PubChem | |
CAS No. |
185017-72-5 | |
Record name | 3-bromo-2-chloro-6-picoline | |
Source | EPA DSSTox | |
URL | https://comptox.epa.gov/dashboard/DTXSID20370297 | |
Description | DSSTox provides a high quality public chemistry resource for supporting improved predictive toxicology. | |
Record name | 3-Bromo-2-chloro-6-methylpyridine | |
Source | European Chemicals Agency (ECHA) | |
URL | https://echa.europa.eu/information-on-chemicals | |
Description | The European Chemicals Agency (ECHA) is an agency of the European Union which is the driving force among regulatory authorities in implementing the EU's groundbreaking chemicals legislation for the benefit of human health and the environment as well as for innovation and competitiveness. | |
Explanation | Use of the information, documents and data from the ECHA website is subject to the terms and conditions of this Legal Notice, and subject to other binding limitations provided for under applicable law, the information, documents and data made available on the ECHA website may be reproduced, distributed and/or used, totally or in part, for non-commercial purposes provided that ECHA is acknowledged as the source: "Source: European Chemicals Agency, http://echa.europa.eu/". Such acknowledgement must be included in each copy of the material. ECHA permits and encourages organisations and individuals to create links to the ECHA website under the following cumulative conditions: Links can only be made to webpages that provide a link to the Legal Notice page. | |
Retrosynthesis Analysis
AI-Powered Synthesis Planning: Our tool employs the Template_relevance Pistachio, Template_relevance Bkms_metabolic, Template_relevance Pistachio_ringbreaker, Template_relevance Reaxys, Template_relevance Reaxys_biocatalysis model, leveraging a vast database of chemical reactions to predict feasible synthetic routes.
One-Step Synthesis Focus: Specifically designed for one-step synthesis, it provides concise and direct routes for your target compounds, streamlining the synthesis process.
Accurate Predictions: Utilizing the extensive PISTACHIO, BKMS_METABOLIC, PISTACHIO_RINGBREAKER, REAXYS, REAXYS_BIOCATALYSIS database, our tool offers high-accuracy predictions, reflecting the latest in chemical research and data.
Strategy Settings
Precursor scoring | Relevance Heuristic |
---|---|
Min. plausibility | 0.01 |
Model | Template_relevance |
Template Set | Pistachio/Bkms_metabolic/Pistachio_ringbreaker/Reaxys/Reaxys_biocatalysis |
Top-N result to add to graph | 6 |
Feasible Synthetic Routes
Disclaimer and Information on In-Vitro Research Products
Please be aware that all articles and product information presented on BenchChem are intended solely for informational purposes. The products available for purchase on BenchChem are specifically designed for in-vitro studies, which are conducted outside of living organisms. In-vitro studies, derived from the Latin term "in glass," involve experiments performed in controlled laboratory settings using cells or tissues. It is important to note that these products are not categorized as medicines or drugs, and they have not received approval from the FDA for the prevention, treatment, or cure of any medical condition, ailment, or disease. We must emphasize that any form of bodily introduction of these products into humans or animals is strictly prohibited by law. It is essential to adhere to these guidelines to ensure compliance with legal and ethical standards in research and experimentation.