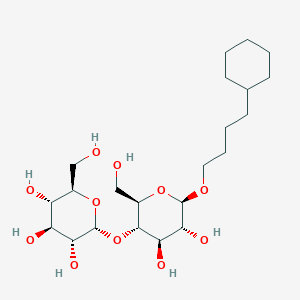
Cymal-4
Overview
Description
GNE 9278 is a positive allosteric modulator of N-methyl-D-aspartate receptors. These receptors are ionotropic glutamate receptors involved in learning, memory, and synaptic plasticity. Dysfunction of these receptors has been implicated in various neurological conditions, such as epilepsy, developmental delay, and ischemic stroke .
Preparation Methods
Synthetic Pathways for Cymal-4
The synthesis of this compound follows a multi-step reaction sequence typical of glycosidic derivatives. While explicit protocols for this compound are scarce, analogous maltoside syntheses provide a framework for its preparation.
Core Reaction Steps
The synthesis begins with the condensation of a cyclohexylalkyl alcohol with a maltose derivative. A hypothetical protocol involves combining cyclohexylbutanol with activated maltose (e.g., maltose peracetate) in a polar aprotic solvent such as dimethylformamide (DMF) or dichloromethane (DCM) . Acid catalysts like p-toluenesulfonic acid (p-TsOH) or Lewis acids (e.g., BF3·Et2O) facilitate glycosidic bond formation. For instance, Matoba et al. demonstrated similar glycosylation using βDDM detergent in buffered conditions, though their work focused on protein solubilization rather than small-molecule synthesis .
Reaction conditions are critical:
-
Temperature : 40–60°C to balance reaction rate and side-product formation.
-
Solvent : Anhydrous DMF ensures solubility of both hydrophilic maltose and hydrophobic cyclohexyl components .
-
Catalyst Loading : 5–10 mol% of p-TsOH achieves optimal conversion without excessive degradation .
Post-reaction, the crude mixture undergoes quenching with aqueous sodium bicarbonate to neutralize acidic catalysts, followed by extraction with ethyl acetate to isolate the product .
Purification and Isolation Techniques
Purifying this compound presents challenges due to its amphiphilic nature, necessitating a combination of chromatographic and crystallization methods.
Column Chromatography
Affinity chromatography using cobalt-based resins, as described in protein purification protocols, offers a potential pathway . In such systems, the resin’s metal ions coordinate with hydroxyl groups on the maltose moiety, enabling selective retention. Elution with imidazole-containing buffers (e.g., 150 mM imidazole in bicine buffer, pH 8.0) displaces the compound while maintaining structural integrity .
Table 1: Chromatographic Purification Parameters
Parameter | Value | Source |
---|---|---|
Resin Type | Cobalt affinity (Talon) | |
Binding Buffer | 20 mM bicine, 500 mM NaCl | |
Elution Buffer | 20 mM bicine, 150 mM imidazole | |
Detergent Concentration | 0.05% βDDM |
Crystallization Optimization
This compound’s crystallization is influenced by polyethylene glycol (PEG) concentrations and buffer pH. Screenings using PEGs (300–4000 MW) in sodium acetate (pH 4.6) or HEPES (pH 7.5) buffers yield hexagonal crystals under vapor-diffusion conditions . For example, a 1:1 mixture of protein solution (10 mg/mL) and reservoir solution (30% PEG 3350, 100 mM MgCl2) produces diffraction-quality crystals .
Advanced Optimization Strategies
Modern organic synthesis leverages high-throughput experimentation (HTE) and machine learning to refine protocols.
High-Throughput Screening
Segmented-flow reactors enable rapid testing of reaction variables. For instance, Bédard et al. optimized photoredox reactions using a modular flow system with in-line analytics, achieving 96 conditions in under an hour . Applying this to this compound synthesis could accelerate catalyst and solvent selection.
Table 2: HTE Parameters for Reaction Optimization
Variable | Range Tested | Source |
---|---|---|
Solvent | DMSO, DMF, pyridine | |
Catalyst | p-TsOH, BF3·Et2O, InCl3 | |
Temperature | 30–70°C | |
Residence Time | 5–30 min |
Machine Learning Integration
Neural networks trained on HTE data predict optimal conditions. Sagmeister et al. demonstrated this in a three-step synthesis, where algorithms processed UV–vis and NMR data to adjust parameters in real time . For this compound, such models could minimize byproducts like hydrolyzed maltose or cyclohexyl dehydration products.
Analytical Characterization
Post-synthesis analysis ensures structural fidelity and purity.
Mass Spectrometry
High-resolution MS (HRMS) confirms the molecular ion at m/z 480.5 [M+H]+, matching the theoretical mass of C22H40O11 . Fragmentation patterns reveal cleavage at glycosidic bonds, consistent with maltoside derivatives.
Nuclear Magnetic Resonance (NMR)
1H NMR (500 MHz, D2O):
-
δ 4.85–4.75 (m, 2H, anomeric protons)
-
δ 3.90–3.30 (m, 38H, maltose and cyclohexyl-O-CH2)
13C NMR confirms glycosidic linkage via anomeric carbon signals at δ 102–105 ppm .
Chemical Reactions Analysis
GNE 9278 undergoes various chemical reactions, including oxidation, reduction, and substitution. Common reagents used in these reactions include oxidizing agents, reducing agents, and nucleophiles. The major products formed from these reactions depend on the specific conditions and reagents used. For example, oxidation of GNE 9278 can lead to the formation of sulfoxides or sulfones, while reduction can result in the formation of amines or alcohols .
Scientific Research Applications
Cymal-4, also known as 4-Cyclohexyl-1-Butyl-β-D-Maltoside, is a non-ionic surfactant that has garnered attention for its applications in various scientific research fields, particularly in biochemistry and structural biology. This article explores the diverse applications of this compound, supported by case studies and comparative data.
Membrane Protein Purification
This compound is widely used for the isolation and purification of membrane proteins. Its effectiveness in solubilizing these proteins makes it a preferred choice over other surfactants like Dodecyl β-D-maltoside (DDM) and Triton X-100, which may be less selective or more toxic .
Case Study: Membrane Protein Extraction
In a study examining various detergents for membrane protein extraction, this compound demonstrated superior performance in maintaining protein activity and stability during purification processes. The study highlighted that this compound could effectively solubilize membrane proteins at concentrations below their critical micelle concentration (CMC), thus minimizing protein aggregation .
Structural Biology
This compound is increasingly utilized in structural biology, particularly in cryo-electron microscopy (cryo-EM) studies. Its ability to extract membrane proteins while preserving their native conformation is essential for high-resolution imaging.
Comparative Analysis of Detergents for Cryo-EM
A comparative study assessed the efficacy of various detergents, including this compound, in preparing samples for cryo-EM. Results indicated that this compound not only solubilized proteins effectively but also reduced the preferred orientation problem often encountered in cryo-EM sample preparation .
Biophysical Studies
This compound plays a significant role in biophysical studies investigating protein interactions and dynamics. Its use allows researchers to explore the kinetics of membrane protein-detergent interactions, providing insights into protein behavior under different conditions.
Kinetic Studies
Research has shown that the kinetics of membrane protein interactions with this compound micelles vary significantly with detergent concentration and pH levels. For instance, experiments revealed that at lower concentrations (1 mM), the desolvation rates were markedly slower compared to higher concentrations (4 mM), indicating a concentration-dependent behavior that is crucial for understanding protein dynamics .
Comparative Data Table
Detergent | Type | Unique Features | Applications |
---|---|---|---|
This compound | Non-Ionic Surfactant | Effective for membrane protein solubilization | Biochemical assays, cryo-EM |
Octyl β-D-glucoside | Non-Ionic Surfactant | Commonly used but less effective than this compound | General protein extraction |
Dodecyl β-D-maltoside | Non-Ionic Surfactant | Higher hydrophobicity; less biocompatible than this compound | Membrane protein studies |
Triton X-100 | Non-Ionic Surfactant | More toxic; less selective for membrane proteins | General laboratory use |
Mechanism of Action
GNE 9278 exerts its effects by binding to the transmembrane domain of N-methyl-D-aspartate receptors. This binding increases the receptor currents in the presence of saturating concentrations of glutamate and glycine. GNE 9278 potentiates N-methyl-D-aspartate receptors containing GluN2A, GluN2B, GluN2C, and GluN2D subunits. The compound slows receptor deactivation and enhances the potency of glutamate and glycine .
Comparison with Similar Compounds
GNE 9278 is unique among positive allosteric modulators of N-methyl-D-aspartate receptors due to its high selectivity and potency. Similar compounds include other positive allosteric modulators, such as GNE-0723 and GNE-0831, which also target N-methyl-D-aspartate receptors but may have different selectivity profiles and potencies . GNE 9278’s ability to potentiate multiple subunits of N-methyl-D-aspartate receptors makes it a valuable tool for research and potential therapeutic applications.
Biological Activity
Cymal-4, a member of the Cymal series of detergents, is recognized for its significant role in the solubilization and stabilization of membrane proteins. This article delves into the biological activity of this compound, highlighting its properties, mechanisms of action, and applications in various research contexts.
Overview of this compound
This compound (4-cyclohexyl-1-butyl-β-D-maltoside) is a zwitterionic detergent that has garnered attention for its efficacy in extracting membrane proteins while maintaining their functional integrity. Its unique structure allows it to interact favorably with various membrane proteins, making it a valuable tool in biochemical and structural biology.
This compound operates through several mechanisms that enhance its biological activity:
- Detergent Properties :
- Protein Interaction :
Case Studies and Experimental Data
Several studies have investigated the effectiveness of this compound in different biological contexts. Below are key findings summarized from recent research:
Biological Applications
This compound has been employed in various applications within biological research:
- Structural Biology :
-
Biochemical Assays :
- Effective in assays aimed at studying protein-protein interactions and enzyme activities, where maintaining protein functionality is crucial.
-
Drug Discovery :
- Plays a role in the pharmaceutical industry for screening potential drug targets that are membrane-bound, facilitating the identification of new therapeutic compounds.
Q & A
Basic Research Questions
Q. How should researchers design experiments to study Cymal-4’s physicochemical properties while ensuring reproducibility?
- Methodological Answer : Begin by defining measurable variables (e.g., solubility, stability under varying pH/temperature). Use controlled experiments with triplicate trials to minimize random errors . For example:
- Independent Variables: Temperature (10°C–50°C), solvent polarity.
- Dependent Variables: Solubility (mg/mL), degradation rate (%/hour).
- Include negative controls (e.g., solvent-only samples) and calibrate instruments (e.g., HPLC) to reduce systematic errors . Document protocols in subsections (e.g., "Synthesis," "Characterization") for clarity .
Q. What statistical methods are appropriate for resolving contradictions in this compound’s reported bioactivity data?
- Methodological Answer : Apply hypothesis testing (e.g., t-tests, ANOVA) to compare datasets. For instance, if Study A reports IC₅₀ = 12 μM (n=3) and Study B reports 25 μM (n=5):
- Calculate effect sizes and confidence intervals to assess significance .
- Use Grubbs’ test to identify outliers in raw data .
- If contradictions persist, conduct meta-analyses to evaluate experimental conditions (e.g., cell lines, assay protocols) as confounding factors .
Q. How can researchers optimize this compound synthesis protocols to improve yield without compromising purity?
- Methodological Answer : Employ a factorial design to test variables (e.g., reaction time, catalyst concentration). Example optimization steps:
Variable | Range Tested | Impact on Yield | Purity (HPLC %) |
---|---|---|---|
Temperature | 60°C–100°C | Positive | Declines >80°C |
Catalyst (mol%) | 1–5 | Peaks at 3% | No change |
- Use response surface methodology (RSM) to identify ideal conditions . Validate purity via NMR and mass spectrometry .
Advanced Research Questions
Q. What strategies address this compound’s solubility limitations in aqueous buffers for in vitro assays?
- Methodological Answer :
- Co-solvent systems : Test DMSO-water gradients (e.g., 5–20% DMSO) and measure solubility via UV-Vis spectroscopy .
- Micellar encapsulation : Use surfactants (e.g., Tween-80) at concentrations below critical micelle concentration (CMC) .
- pH adjustment : Conduct solubility assays across pH 4–9 to identify optimal ionization states . Report solubility curves with error bars from triplicate trials .
Q. How can advanced spectroscopic techniques elucidate this compound’s interaction with lipid bilayers?
- Methodological Answer :
- Fluorescence quenching : Incorporate this compound into liposomes with pyrene-labeled lipids; measure emission spectra shifts .
- NMR dynamics : Use ¹H-NMR to monitor chemical shift changes in lipid headgroups upon this compound binding .
- Molecular dynamics (MD) simulations : Cross-validate experimental data with MD models to predict partition coefficients .
Q. What frameworks reconcile contradictory findings in this compound’s role as a membrane protein stabilizer versus disruptor?
- Methodological Answer :
- Contextual analysis : Compare studies for differences in protein type (e.g., GPCRs vs. ion channels) and lipid composition .
- Dose-response profiling : Replicate experiments across a broader concentration range (e.g., 0.1–50 μM) to identify biphasic effects .
- Structural analogs : Test this compound derivatives to isolate functional groups responsible for stabilization/disruption .
Q. How should researchers evaluate long-term stability of this compound in storage conditions relevant to academic labs?
- Methodological Answer :
- Design accelerated stability studies (e.g., 40°C/75% RH for 6 months) and monitor degradation via LC-MS .
- Use Arrhenius equation to extrapolate shelf life at 4°C .
- Compare lyophilized vs. solution storage; include desiccants for solid forms .
Q. Data Presentation and Validation
-
Table 1 : Example stability data for this compound under varying conditions:
Condition Degradation Rate (%/month) Major Degradant Identified 4°C (solution) 0.8 ± 0.2 None 25°C (lyophilized) 0.3 ± 0.1 Oxidation product 40°C/75% RH 5.1 ± 1.0 Hydrolyzed derivative -
Key Considerations :
Properties
IUPAC Name |
(2R,3R,4S,5S,6R)-2-[(2R,3S,4R,5R,6R)-6-(4-cyclohexylbutoxy)-4,5-dihydroxy-2-(hydroxymethyl)oxan-3-yl]oxy-6-(hydroxymethyl)oxane-3,4,5-triol | |
---|---|---|
Source | PubChem | |
URL | https://pubchem.ncbi.nlm.nih.gov | |
Description | Data deposited in or computed by PubChem | |
InChI |
InChI=1S/C22H40O11/c23-10-13-15(25)16(26)18(28)22(31-13)33-20-14(11-24)32-21(19(29)17(20)27)30-9-5-4-8-12-6-2-1-3-7-12/h12-29H,1-11H2/t13-,14-,15-,16+,17-,18-,19-,20-,21-,22-/m1/s1 | |
Source | PubChem | |
URL | https://pubchem.ncbi.nlm.nih.gov | |
Description | Data deposited in or computed by PubChem | |
InChI Key |
JRNQXDHDSXBSFV-WXFJLFHKSA-N | |
Source | PubChem | |
URL | https://pubchem.ncbi.nlm.nih.gov | |
Description | Data deposited in or computed by PubChem | |
Canonical SMILES |
C1CCC(CC1)CCCCOC2C(C(C(C(O2)CO)OC3C(C(C(C(O3)CO)O)O)O)O)O | |
Source | PubChem | |
URL | https://pubchem.ncbi.nlm.nih.gov | |
Description | Data deposited in or computed by PubChem | |
Isomeric SMILES |
C1CCC(CC1)CCCCO[C@H]2[C@@H]([C@H]([C@@H]([C@H](O2)CO)O[C@@H]3[C@@H]([C@H]([C@@H]([C@H](O3)CO)O)O)O)O)O | |
Source | PubChem | |
URL | https://pubchem.ncbi.nlm.nih.gov | |
Description | Data deposited in or computed by PubChem | |
Molecular Formula |
C22H40O11 | |
Source | PubChem | |
URL | https://pubchem.ncbi.nlm.nih.gov | |
Description | Data deposited in or computed by PubChem | |
DSSTOX Substance ID |
DTXSID50470113 | |
Record name | 4-Cyclohexylbutyl-4-O-(a-D-glucopyranosyl)-b-D-glucopyranoside | |
Source | EPA DSSTox | |
URL | https://comptox.epa.gov/dashboard/DTXSID50470113 | |
Description | DSSTox provides a high quality public chemistry resource for supporting improved predictive toxicology. | |
Molecular Weight |
480.5 g/mol | |
Source | PubChem | |
URL | https://pubchem.ncbi.nlm.nih.gov | |
Description | Data deposited in or computed by PubChem | |
CAS No. |
181135-57-9 | |
Record name | 4-Cyclohexylbutyl-4-O-(a-D-glucopyranosyl)-b-D-glucopyranoside | |
Source | EPA DSSTox | |
URL | https://comptox.epa.gov/dashboard/DTXSID50470113 | |
Description | DSSTox provides a high quality public chemistry resource for supporting improved predictive toxicology. | |
Disclaimer and Information on In-Vitro Research Products
Please be aware that all articles and product information presented on BenchChem are intended solely for informational purposes. The products available for purchase on BenchChem are specifically designed for in-vitro studies, which are conducted outside of living organisms. In-vitro studies, derived from the Latin term "in glass," involve experiments performed in controlled laboratory settings using cells or tissues. It is important to note that these products are not categorized as medicines or drugs, and they have not received approval from the FDA for the prevention, treatment, or cure of any medical condition, ailment, or disease. We must emphasize that any form of bodily introduction of these products into humans or animals is strictly prohibited by law. It is essential to adhere to these guidelines to ensure compliance with legal and ethical standards in research and experimentation.