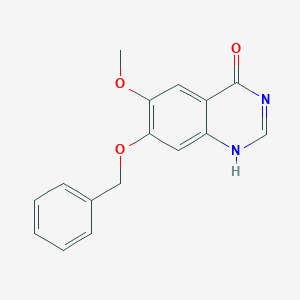
7-(benzyloxy)-6-methoxyquinazolin-4(3H)-one
Overview
Description
7-Benzyloxy-6-methoxy-3H-quinazolin-4-one is a synthetic organic compound with the molecular formula C16H14N2O3 and a molecular weight of 282.3 g/mol . It is a derivative of quinazolinone, a class of compounds known for their diverse biological activities. This compound is characterized by the presence of a benzyloxy group at the 7th position and a methoxy group at the 6th position on the quinazolinone core .
Preparation Methods
Synthetic Routes and Reaction Conditions
The synthesis of 7-Benzyloxy-6-methoxy-3H-quinazolin-4-one typically involves the following steps:
Starting Materials: The synthesis begins with the appropriate substituted aniline and benzyl bromide.
Formation of Intermediate: The aniline derivative undergoes a nucleophilic substitution reaction with benzyl bromide to form the benzyloxy intermediate.
Cyclization: The intermediate is then subjected to cyclization under acidic or basic conditions to form the quinazolinone core.
Methoxylation: Finally, the compound is methoxylated at the 6th position using a suitable methylating agent such as dimethyl sulfate or methyl iodide.
Industrial Production Methods
Industrial production of 7-Benzyloxy-6-methoxy-3H-quinazolin-4-one follows similar synthetic routes but on a larger scale. The process involves optimization of reaction conditions to ensure high yield and purity. Common techniques include:
Batch Processing: Reactions are carried out in large reactors with precise control over temperature, pressure, and reaction time.
Purification: The product is purified using techniques such as recrystallization, chromatography, or distillation to achieve the desired purity.
Chemical Reactions Analysis
Types of Reactions
7-Benzyloxy-6-methoxy-3H-quinazolin-4-one undergoes various chemical reactions, including:
Oxidation: The compound can be oxidized using oxidizing agents such as potassium permanganate or chromium trioxide to form quinazolinone derivatives with different oxidation states.
Reduction: Reduction reactions using reducing agents like lithium aluminum hydride can convert the quinazolinone core to its corresponding dihydroquinazoline derivative.
Substitution: The benzyloxy and methoxy groups can be substituted with other functional groups using nucleophilic substitution reactions.
Common Reagents and Conditions
Oxidation: Potassium permanganate in acidic medium.
Reduction: Lithium aluminum hydride in anhydrous ether.
Substitution: Sodium hydride in dimethylformamide for nucleophilic substitution.
Major Products Formed
Oxidation: Quinazolinone derivatives with higher oxidation states.
Reduction: Dihydroquinazoline derivatives.
Substitution: Compounds with different functional groups replacing the benzyloxy or methoxy groups.
Scientific Research Applications
7-Benzyloxy-6-methoxy-3H-quinazolin-4-one has several applications in scientific research:
Chemistry: Used as a building block in the synthesis of more complex quinazolinone derivatives.
Biology: Studied for its potential biological activities, including antimicrobial, anticancer, and anti-inflammatory properties.
Medicine: Investigated for its potential as a therapeutic agent in the treatment of various diseases.
Industry: Utilized in the development of new materials and chemical processes
Mechanism of Action
The mechanism of action of 7-Benzyloxy-6-methoxy-3H-quinazolin-4-one involves its interaction with specific molecular targets and pathways:
Molecular Targets: The compound may interact with enzymes, receptors, or other proteins involved in cellular processes.
Pathways Involved: It can modulate signaling pathways related to cell proliferation, apoptosis, and inflammation.
Comparison with Similar Compounds
Similar Compounds
- 7-Methoxy-6-benzyloxyquinazolin-4-one
- 7-Methoxy-6-(3-morpholin-4-ylpropoxy)quinazolin-4-one
- 4-Hydroxyquinazoline
Uniqueness
7-Benzyloxy-6-methoxy-3H-quinazolin-4-one is unique due to its specific substitution pattern, which imparts distinct chemical and biological properties. Compared to similar compounds, it may exhibit different reactivity and biological activity profiles .
Properties
IUPAC Name |
6-methoxy-7-phenylmethoxy-3H-quinazolin-4-one | |
---|---|---|
Source | PubChem | |
URL | https://pubchem.ncbi.nlm.nih.gov | |
Description | Data deposited in or computed by PubChem | |
InChI |
InChI=1S/C16H14N2O3/c1-20-14-7-12-13(17-10-18-16(12)19)8-15(14)21-9-11-5-3-2-4-6-11/h2-8,10H,9H2,1H3,(H,17,18,19) | |
Source | PubChem | |
URL | https://pubchem.ncbi.nlm.nih.gov | |
Description | Data deposited in or computed by PubChem | |
InChI Key |
ZCUFFSHMOAEEIL-UHFFFAOYSA-N | |
Source | PubChem | |
URL | https://pubchem.ncbi.nlm.nih.gov | |
Description | Data deposited in or computed by PubChem | |
Canonical SMILES |
COC1=C(C=C2C(=C1)C(=O)NC=N2)OCC3=CC=CC=C3 | |
Source | PubChem | |
URL | https://pubchem.ncbi.nlm.nih.gov | |
Description | Data deposited in or computed by PubChem | |
Molecular Formula |
C16H14N2O3 | |
Source | PubChem | |
URL | https://pubchem.ncbi.nlm.nih.gov | |
Description | Data deposited in or computed by PubChem | |
DSSTOX Substance ID |
DTXSID00441530 | |
Record name | 7-(Benzyloxy)-6-methoxyquinazolin-4(1H)-one | |
Source | EPA DSSTox | |
URL | https://comptox.epa.gov/dashboard/DTXSID00441530 | |
Description | DSSTox provides a high quality public chemistry resource for supporting improved predictive toxicology. | |
Molecular Weight |
282.29 g/mol | |
Source | PubChem | |
URL | https://pubchem.ncbi.nlm.nih.gov | |
Description | Data deposited in or computed by PubChem | |
CAS No. |
179688-01-8 | |
Record name | 7-(Benzyloxy)-6-methoxyquinazolin-4(1H)-one | |
Source | EPA DSSTox | |
URL | https://comptox.epa.gov/dashboard/DTXSID00441530 | |
Description | DSSTox provides a high quality public chemistry resource for supporting improved predictive toxicology. | |
Record name | 7-Benzyloxy-6-methoxy-3H-quinazolin-4-one | |
Source | European Chemicals Agency (ECHA) | |
URL | https://echa.europa.eu/information-on-chemicals | |
Description | The European Chemicals Agency (ECHA) is an agency of the European Union which is the driving force among regulatory authorities in implementing the EU's groundbreaking chemicals legislation for the benefit of human health and the environment as well as for innovation and competitiveness. | |
Explanation | Use of the information, documents and data from the ECHA website is subject to the terms and conditions of this Legal Notice, and subject to other binding limitations provided for under applicable law, the information, documents and data made available on the ECHA website may be reproduced, distributed and/or used, totally or in part, for non-commercial purposes provided that ECHA is acknowledged as the source: "Source: European Chemicals Agency, http://echa.europa.eu/". Such acknowledgement must be included in each copy of the material. ECHA permits and encourages organisations and individuals to create links to the ECHA website under the following cumulative conditions: Links can only be made to webpages that provide a link to the Legal Notice page. | |
Retrosynthesis Analysis
AI-Powered Synthesis Planning: Our tool employs the Template_relevance Pistachio, Template_relevance Bkms_metabolic, Template_relevance Pistachio_ringbreaker, Template_relevance Reaxys, Template_relevance Reaxys_biocatalysis model, leveraging a vast database of chemical reactions to predict feasible synthetic routes.
One-Step Synthesis Focus: Specifically designed for one-step synthesis, it provides concise and direct routes for your target compounds, streamlining the synthesis process.
Accurate Predictions: Utilizing the extensive PISTACHIO, BKMS_METABOLIC, PISTACHIO_RINGBREAKER, REAXYS, REAXYS_BIOCATALYSIS database, our tool offers high-accuracy predictions, reflecting the latest in chemical research and data.
Strategy Settings
Precursor scoring | Relevance Heuristic |
---|---|
Min. plausibility | 0.01 |
Model | Template_relevance |
Template Set | Pistachio/Bkms_metabolic/Pistachio_ringbreaker/Reaxys/Reaxys_biocatalysis |
Top-N result to add to graph | 6 |
Feasible Synthetic Routes
Q1: Why is the analysis of 6-Methoxy-7-benzyloxyquinazolin-4-one and its related substances important?
A1: 6-Methoxy-7-benzyloxyquinazolin-4-one serves as a key starting material in the multi-step synthesis of Vandetanib []. The quality and purity of this compound directly influence the quality of the final drug product. Therefore, having a robust analytical method, such as the HPLC method described in the research [, ], to identify and quantify this compound and any potential impurities is critical for ensuring the production of safe and effective Vandetanib.
Q2: What analytical method has been developed for the analysis of 6-Methoxy-7-benzyloxyquinazolin-4-one?
A2: Researchers have successfully developed and validated a High-Performance Liquid Chromatography (HPLC) method for the determination of 6-Methoxy-7-benzyloxyquinazolin-4-one and its related substances [, ]. The method utilizes an Agela C18 column with a gradient elution using a mobile phase of 0.01 mol/L potassium dihydrogen phosphate solution (pH 3.0) and methanol. Detection is carried out at a wavelength of 240 nm, achieving a low detection limit of 11 ng []. This method effectively separates the target compound from its potential impurities, including key intermediates and degradation products [].
Disclaimer and Information on In-Vitro Research Products
Please be aware that all articles and product information presented on BenchChem are intended solely for informational purposes. The products available for purchase on BenchChem are specifically designed for in-vitro studies, which are conducted outside of living organisms. In-vitro studies, derived from the Latin term "in glass," involve experiments performed in controlled laboratory settings using cells or tissues. It is important to note that these products are not categorized as medicines or drugs, and they have not received approval from the FDA for the prevention, treatment, or cure of any medical condition, ailment, or disease. We must emphasize that any form of bodily introduction of these products into humans or animals is strictly prohibited by law. It is essential to adhere to these guidelines to ensure compliance with legal and ethical standards in research and experimentation.