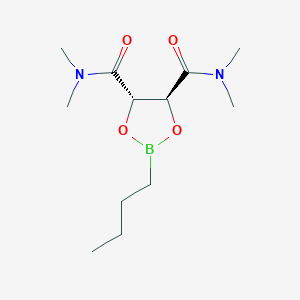
(4S,5S)-2-Butyl-N4,N4,N5,N5-tetramethyl-1,3,2-dioxaborolane-4,5-dicarboxamide
Overview
Description
(4S,5S)-2-Butyl-N4,N4,N5,N5-tetramethyl-1,3,2-dioxaborolane-4,5-dicarboxamide is a boron-containing compound that features a dioxaborolane ring. Compounds with boron atoms are of significant interest in various fields of chemistry due to their unique properties and reactivity.
Preparation Methods
Synthetic Routes and Reaction Conditions
The synthesis of (4S,5S)-2-Butyl-N4,N4,N5,N5-tetramethyl-1,3,2-dioxaborolane-4,5-dicarboxamide typically involves the formation of the dioxaborolane ring followed by the introduction of the butyl and tetramethyl groups. Common synthetic routes may include:
Formation of the Dioxaborolane Ring: This can be achieved through the reaction of boronic acids or boronates with diols under acidic or basic conditions.
Introduction of Butyl and Tetramethyl Groups: Alkylation reactions using butyl halides and methylating agents such as methyl iodide or dimethyl sulfate.
Industrial Production Methods
Industrial production methods for such compounds often involve large-scale reactions in batch or continuous flow reactors. The choice of solvents, catalysts, and purification techniques are optimized for yield and purity.
Chemical Reactions Analysis
Types of Reactions
Oxidation: The compound may undergo oxidation reactions, particularly at the boron center, leading to the formation of boronic acids or borates.
Reduction: Reduction reactions can convert the boron center to different oxidation states, potentially forming borohydrides.
Substitution: Nucleophilic or electrophilic substitution reactions can occur at the carbon or nitrogen atoms, leading to various derivatives.
Common Reagents and Conditions
Oxidation: Hydrogen peroxide, oxygen, or other oxidizing agents.
Reduction: Sodium borohydride, lithium aluminum hydride.
Substitution: Halides, alkylating agents, or nucleophiles.
Major Products
The major products of these reactions depend on the specific conditions and reagents used. For example, oxidation may yield boronic acids, while substitution reactions can produce a variety of functionalized derivatives.
Scientific Research Applications
Chemistry
In chemistry, (4S,5S)-2-Butyl-N4,N4,N5,N5-tetramethyl-1,3,2-dioxaborolane-4,5-dicarboxamide can be used as a reagent or catalyst in organic synthesis, particularly in the formation of carbon-boron bonds.
Biology
In biological research, boron-containing compounds are explored for their potential as enzyme inhibitors or as tools for studying boron metabolism in organisms.
Medicine
Medicinal applications may include the development of boron-based drugs for cancer therapy, as boron neutron capture therapy (BNCT) relies on the unique properties of boron atoms.
Industry
In industry, such compounds can be used in materials science for the development of boron-containing polymers or as additives in lubricants and coatings.
Mechanism of Action
The mechanism of action of (4S,5S)-2-Butyl-N4,N4,N5,N5-tetramethyl-1,3,2-dioxaborolane-4,5-dicarboxamide involves interactions with molecular targets such as enzymes or receptors. The boron atom can form reversible covalent bonds with nucleophilic sites, affecting the activity of biological molecules.
Comparison with Similar Compounds
Similar Compounds
Boronic Acids: Compounds with a boron-oxygen bond, commonly used in organic synthesis.
Borates: Salts or esters of boric acid, used in various industrial applications.
Borohydrides: Compounds containing boron-hydrogen bonds, used as reducing agents.
Uniqueness
(4S,5S)-2-Butyl-N4,N4,N5,N5-tetramethyl-1,3,2-dioxaborolane-4,5-dicarboxamide is unique due to its specific dioxaborolane ring structure and the presence of butyl and tetramethyl groups, which may confer distinct reactivity and properties compared to other boron-containing compounds.
For precise and detailed information, consulting specialized chemical databases or scientific literature is recommended.
Biological Activity
(4S,5S)-2-Butyl-N4,N4,N5,N5-tetramethyl-1,3,2-dioxaborolane-4,5-dicarboxamide, commonly referred to as dioxaborolane, is a boron-containing compound with significant implications in biological and medicinal chemistry. This article explores its biological activity, focusing on its mechanisms of action, therapeutic potential, and relevant case studies.
- CAS Number : 161344-84-9
- Molecular Formula : C12H23BN2O4
- Molecular Weight : 270.133 g/mol
- Purity : 95%
Property | Value |
---|---|
Molecular Weight | 270.133 g/mol |
Purity | 95% |
CAS Number | 161344-84-9 |
MDL Number | MFCD05663838 |
The biological activity of dioxaborolane is primarily attributed to its ability to interact with various biomolecules through boron coordination chemistry. The unique structure allows it to participate in:
- Enzyme Inhibition : Dioxaborolanes can inhibit enzymes by forming stable complexes with active site residues, thereby altering enzyme activity and affecting metabolic pathways.
- Gene Regulation : Some studies suggest that dioxaborolanes can modulate gene expression by interacting with DNA or RNA structures.
- Cell Signaling : The compound may influence cell signaling pathways by acting as a ligand for specific receptors.
Anticancer Properties
Research indicates that (4S,5S)-2-butyl-dioxaborolane exhibits anticancer activity through several mechanisms:
- Apoptosis Induction : The compound has been shown to induce apoptosis in various cancer cell lines by activating caspase pathways.
- Cell Cycle Arrest : It can halt the cell cycle at specific checkpoints, preventing cancer cells from proliferating.
Case Study: Breast Cancer Cells
In a study involving breast cancer cell lines (MCF-7), treatment with dioxaborolane resulted in a significant reduction in cell viability (up to 70% at concentrations of 50 µM) compared to controls. Flow cytometry analysis revealed an increase in apoptotic cells post-treatment.
Antimicrobial Activity
Dioxaborolane also demonstrates antimicrobial properties against various pathogens:
- Bacterial Inhibition : It has shown effectiveness against Gram-positive bacteria such as Staphylococcus aureus and Streptococcus pneumoniae.
- Fungal Activity : Preliminary data suggest activity against common fungal strains.
Case Study: Antibacterial Efficacy
A study evaluated the minimum inhibitory concentration (MIC) of dioxaborolane against E. coli. Results indicated an MIC of 32 µg/mL, suggesting potential as an antibacterial agent.
Pharmacokinetics and Toxicity
Understanding the pharmacokinetics of dioxaborolane is crucial for its therapeutic application:
- Absorption and Distribution : Studies indicate that dioxaborolane is well absorbed when administered orally and distributed widely in tissues.
- Metabolism : The compound undergoes metabolic conversion primarily in the liver.
- Excretion : Renal excretion is the primary route for elimination.
Toxicity studies have shown that while dioxaborolane exhibits low acute toxicity (LD50 > 2000 mg/kg in rodents), chronic exposure may lead to reproductive and developmental effects.
Properties
IUPAC Name |
(4S,5S)-2-butyl-4-N,4-N,5-N,5-N-tetramethyl-1,3,2-dioxaborolane-4,5-dicarboxamide | |
---|---|---|
Source | PubChem | |
URL | https://pubchem.ncbi.nlm.nih.gov | |
Description | Data deposited in or computed by PubChem | |
InChI |
InChI=1S/C12H23BN2O4/c1-6-7-8-13-18-9(11(16)14(2)3)10(19-13)12(17)15(4)5/h9-10H,6-8H2,1-5H3/t9-,10-/m0/s1 | |
Source | PubChem | |
URL | https://pubchem.ncbi.nlm.nih.gov | |
Description | Data deposited in or computed by PubChem | |
InChI Key |
AFQWQRBBIZKYTE-UWVGGRQHSA-N | |
Source | PubChem | |
URL | https://pubchem.ncbi.nlm.nih.gov | |
Description | Data deposited in or computed by PubChem | |
Canonical SMILES |
B1(OC(C(O1)C(=O)N(C)C)C(=O)N(C)C)CCCC | |
Source | PubChem | |
URL | https://pubchem.ncbi.nlm.nih.gov | |
Description | Data deposited in or computed by PubChem | |
Isomeric SMILES |
B1(O[C@@H]([C@H](O1)C(=O)N(C)C)C(=O)N(C)C)CCCC | |
Source | PubChem | |
URL | https://pubchem.ncbi.nlm.nih.gov | |
Description | Data deposited in or computed by PubChem | |
Molecular Formula |
C12H23BN2O4 | |
Source | PubChem | |
URL | https://pubchem.ncbi.nlm.nih.gov | |
Description | Data deposited in or computed by PubChem | |
DSSTOX Substance ID |
DTXSID20450583 | |
Record name | (4S,5S)-2-Butyl-N~4~,N~4~,N~5~,N~5~-tetramethyl-1,3,2-dioxaborolane-4,5-dicarboxamide | |
Source | EPA DSSTox | |
URL | https://comptox.epa.gov/dashboard/DTXSID20450583 | |
Description | DSSTox provides a high quality public chemistry resource for supporting improved predictive toxicology. | |
Molecular Weight |
270.14 g/mol | |
Source | PubChem | |
URL | https://pubchem.ncbi.nlm.nih.gov | |
Description | Data deposited in or computed by PubChem | |
CAS No. |
161344-84-9 | |
Record name | (4S,5S)-2-Butyl-N~4~,N~4~,N~5~,N~5~-tetramethyl-1,3,2-dioxaborolane-4,5-dicarboxamide | |
Source | EPA DSSTox | |
URL | https://comptox.epa.gov/dashboard/DTXSID20450583 | |
Description | DSSTox provides a high quality public chemistry resource for supporting improved predictive toxicology. | |
Disclaimer and Information on In-Vitro Research Products
Please be aware that all articles and product information presented on BenchChem are intended solely for informational purposes. The products available for purchase on BenchChem are specifically designed for in-vitro studies, which are conducted outside of living organisms. In-vitro studies, derived from the Latin term "in glass," involve experiments performed in controlled laboratory settings using cells or tissues. It is important to note that these products are not categorized as medicines or drugs, and they have not received approval from the FDA for the prevention, treatment, or cure of any medical condition, ailment, or disease. We must emphasize that any form of bodily introduction of these products into humans or animals is strictly prohibited by law. It is essential to adhere to these guidelines to ensure compliance with legal and ethical standards in research and experimentation.