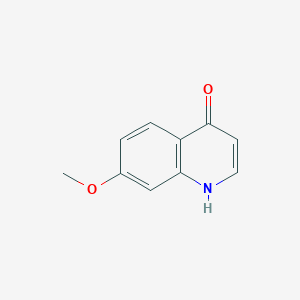
4-Hydroxy-7-methoxyquinoline
Overview
Description
4-Hydroxy-7-methoxyquinoline (CAS 82121-05-9) is a quinoline derivative with the molecular formula C₁₀H₉NO₂ and a molecular weight of 175.18 g/mol . It exists as a solid under standard conditions and is stored at room temperature in airtight containers to avoid degradation . The compound features a hydroxyl group at the 4-position and a methoxy group at the 7-position of the quinoline backbone (Figure 1). It is used in pharmaceutical and biochemical research, particularly in studies related to tumor suppression, apoptosis, and kinase signaling pathways .
Preparation Methods
Alternative Synthesis Using Trimethyl Orthoformate and Diphenyl Ether
A 2020 patent (CN111440118A) describes a modified approach that substitutes triethyl orthoformate with trimethyl orthoformate and employs diphenyl ether as a high-boiling solvent to reduce energy consumption.
Reaction Optimization
The process begins with the reaction of trimethyl orthoformate and isopropylidene malonate at 60–65°C, followed by the addition of 3,4-dimethoxyaniline. Refluxing at 170–180°C in diphenyl ether facilitates cyclization, with strict temperature control (±5°C) to prevent decomposition. This method achieves a total yield of 75.3–79.3% , attributed to the recycling of trimethyl orthoformate, which reduces raw material costs by 15–20% .
Solvent and Temperature Effects
Diphenyl ether’s high boiling point (258°C) enables reactions at elevated temperatures without rapid solvent evaporation. Comparative studies show that replacing diphenyl ether with toluene or xylene lowers yields by 12–18% due to incomplete cyclization .
Purification via Dichloromethane Extraction
The crude product is stirred with dichloromethane (1:1 mass ratio) at room temperature, effectively removing non-polar byproducts. Centrifugation and vacuum drying yield a final product with 99.4% purity, as confirmed by gas chromatography .
Industrial-Scale Production and Quality Control
Industrial methods prioritize scalability and compliance with pharmaceutical regulations.
Precursor Synthesis and Coupling Reactions
Large-scale synthesis (2000L reactors) involves:
-
Williamson ether synthesis to generate 7-methoxyquinoline precursors.
-
Michael addition for coupling 4-hydroxyquinoline and methoxy-substituted intermediates .
Hydrogen peroxide and sodium hydroxide are used to facilitate oxidative coupling, though yields are sensitive to pH (optimal range: 10.5–11.5) .
Chromatographic Purification
High-performance liquid chromatography (HPLC) is employed for final purification, with C18 reverse-phase columns resolving this compound from structurally similar contaminants. Process parameters include:
Parameter | Value |
---|---|
Mobile phase | Acetonitrile/water (70:30) |
Flow rate | 1.5 mL/min |
Column temperature | 25°C |
Retention time | 8.2 min |
This step ensures >99.5% purity, meeting pharmacopeial standards .
Regulatory Compliance and Stability Testing
Stability studies under ICH guidelines (25°C/60% RH) confirm a shelf life of 24 months for the purified compound. Accelerated degradation tests (40°C/75% RH) show no significant loss of potency over 6 months .
Comparative Analysis of Synthesis Methods
Method | Yield (%) | Purity (%) | Key Advantage | Limitation |
---|---|---|---|---|
Triethyl orthoformate | 78–82 | >99 | High yield, established protocol | High energy consumption |
Trimethyl orthoformate | 75–79 | 99.4 | Solvent recycling, cost-effective | Longer reaction times |
Industrial-scale | 70–75 | >99.5 | Regulatory compliance | Requires specialized equipment |
Chemical Reactions Analysis
Types of Reactions: 4-Hydroxy-7-methoxyquinoline undergoes various chemical reactions, including:
Oxidation: This reaction can be facilitated by oxidizing agents such as potassium permanganate or hydrogen peroxide.
Reduction: Reduction reactions can be carried out using reducing agents like sodium borohydride or lithium aluminum hydride.
Substitution: The compound can participate in substitution reactions, where functional groups are replaced by other groups under specific conditions.
Common Reagents and Conditions:
Oxidation: Potassium permanganate in an acidic medium.
Reduction: Sodium borohydride in methanol.
Substitution: Halogenation using chlorine or bromine in the presence of a catalyst.
Major Products Formed:
Oxidation: Formation of quinoline derivatives with additional oxygen-containing functional groups.
Reduction: Formation of reduced quinoline derivatives.
Substitution: Formation of halogenated quinoline derivatives.
Scientific Research Applications
4-Hydroxy-7-methoxyquinoline has a wide range of applications in scientific research:
Chemistry: Used as a building block for the synthesis of more complex molecules.
Biology: Investigated for its potential biological activities, including antimicrobial and anticancer properties.
Medicine: Explored for its therapeutic potential in treating various diseases.
Industry: Utilized in the development of dyes, pigments, and other industrial chemicals
Mechanism of Action
The mechanism of action of 4-Hydroxy-7-methoxyquinoline involves its interaction with specific molecular targets and pathways. It can act as an inhibitor or modulator of certain enzymes and receptors, leading to various biological effects. The exact molecular targets and pathways depend on the specific application and context of use .
Comparison with Similar Compounds
Quinoline derivatives exhibit diverse biological and chemical properties depending on substituent positions and functional groups. Below is a detailed comparison of 4-Hydroxy-7-methoxyquinoline with structurally or functionally related compounds.
Structural Analogs with Positional Isomerism
Key Observations :
- Functional Group Impact: Chlorination at the 4-position (e.g., 4-Chloro-6,7-dimethoxyquinoline) increases electrophilicity, making the compound more reactive in coupling reactions .
Derivatives with Modified Functional Groups
Key Observations :
- Carboxylic Acid Derivatives: 7-Methoxyquinoline-4-carboxylic acid shows high structural similarity (96%) to this compound but introduces a carboxyl group, enhancing polarity and metal-binding capacity .
Key Observations :
- Toxicity: Ethoxy-substituted quinolines (e.g., 7-(2-ethoxyethoxy)quinoline) exhibit higher acute toxicity than hydroxyl/methoxy analogs, likely due to increased lipophilicity .
- Bioactivity: Chlorinated derivatives (e.g., 4-Chloro-6,7-dimethoxyquinoline) are less explored for direct biological activity but serve as key intermediates for antimicrobial agents .
Reactivity Trends :
- Electrophilic Substitution: The 3-position of this compound is reactive toward bromination, enabling further derivatization .
- Nucleophilic Displacement: Chlorinated quinolines undergo nucleophilic substitution with amines or alkoxides to form bioactive molecules .
Biological Activity
4-Hydroxy-7-methoxyquinoline (4-H7MQ) is a quinoline derivative that has garnered attention in various fields, particularly for its potential biological activities. This article explores its biochemical properties, mechanisms of action, and therapeutic applications, supported by relevant data and case studies.
Chemical Structure and Properties
This compound has the molecular formula C_10H_9NO_2 and a molecular weight of 175.18 g/mol. The compound features a quinoline ring structure with hydroxyl (-OH) and methoxy (-OCH₃) functional groups at the 4 and 7 positions, respectively. This unique substitution pattern significantly influences its chemical reactivity and biological properties.
Property | Value |
---|---|
Molecular Formula | C₁₀H₉NO₂ |
Molecular Weight | 175.18 g/mol |
Functional Groups | Hydroxyl, Methoxy |
Structural Features | Quinoline ring |
Antimicrobial Properties
Research indicates that 4-H7MQ exhibits notable antimicrobial activity against various bacterial strains. A study highlighted its effectiveness against Gram-positive bacteria such as Bacillus subtilis and Staphylococcus aureus, with minimum inhibitory concentrations (MICs) reported as low as 8 µg/mL for certain derivatives . These findings suggest that 4-H7MQ could potentially serve as a lead compound in developing new antibacterial agents.
Anticancer Activity
The anticancer potential of 4-H7MQ has been explored through various studies. One significant investigation revealed that derivatives of 4-H7MQ displayed selective cytotoxicity towards resistant cancer cell lines compared to doxorubicin-sensitive ones. The selective toxicity observed may lead to reduced side effects in normal cells, making it a promising candidate for targeted cancer therapies .
Table: Cytotoxic Effects on Cancer Cell Lines
Compound | Cell Line Type | Selectivity | MIC (µg/mL) |
---|---|---|---|
Derivative A | Doxorubicin-sensitive | Low | N/A |
Derivative B | Doxorubicin-resistant | High | 16 |
Derivative C | Normal human fibroblasts | Low | N/A |
The mechanism by which 4-H7MQ exerts its biological effects involves several biochemical interactions:
- Enzyme Inhibition : It is believed to inhibit specific enzymes involved in cellular processes, thereby altering metabolic pathways.
- DNA Interaction : Preliminary studies suggest that it may interact with DNA, affecting gene expression and cellular signaling pathways.
- Cellular Metabolism : The compound may influence cellular metabolism by interacting with various cofactors and enzymes, impacting overall cell function.
Case Studies
- Antibacterial Efficacy : A study evaluated the antibacterial efficacy of several derivatives of 4-H7MQ against common pathogens. The results indicated that certain derivatives had enhanced activity against Gram-positive bacteria compared to traditional antibiotics like penicillin G .
- Cytotoxicity in Cancer Research : In a comparative study involving colon adenocarcinoma cell lines, some derivatives showed significant selective toxicity against resistant cells. This selectivity is crucial for minimizing adverse effects on normal tissues during cancer treatment .
Q & A
Basic Research Questions
Q. What are the standard synthetic routes for 4-Hydroxy-7-methoxyquinoline, and how do reaction conditions influence yield?
- Methodological Answer : The compound is commonly synthesized via bromination of this compound using N-bromosuccinimide (NBS) in DMF at 55°C, yielding 3-bromo-7-methoxyquinolin-4-ol quantitatively . Optimization involves controlling stoichiometry (e.g., 1.2 equiv NBS) and reaction time. Alternative routes include chlorination with POCl₃, though intermediates may require purification via column chromatography . For regioselective functionalization, reflux conditions with sodium hydroxide in methanol/water mixtures are recommended to avoid side reactions .
Q. How can researchers validate the purity of this compound derivatives post-synthesis?
- Methodological Answer : Use a combination of HPLC (reverse-phase C18 column, acetonitrile/water gradient) and ¹H/¹³C NMR to confirm structural integrity. For example, the absence of characterization in bromination intermediates (e.g., 3-bromo-7-methoxyquinolin-4-ol) highlights the need for pre-functionalization validation to avoid downstream impurities . Mass spectrometry (ESI-MS) is critical for verifying molecular weights, especially for halogenated derivatives .
Advanced Research Questions
Q. What strategies address contradictions in regioselectivity during quinoline functionalization?
- Methodological Answer : Discrepancies in substitution patterns (e.g., bromination at C3 vs. C5) can arise from solvent polarity and catalyst choice. For this compound, DMF promotes electrophilic aromatic substitution at the C3 position due to its high polarity stabilizing transition states . Computational modeling (DFT) of charge distribution in the quinoline ring can predict reactive sites, while kinetic studies under varying temperatures resolve competing pathways .
Q. How do methoxy and hydroxyl substituents influence the compound’s electronic properties and biological activity?
- Methodological Answer : The electron-donating methoxy group at C7 increases ring electron density, enhancing interactions with biological targets (e.g., enzymes or DNA). Comparative studies with analogues like 6,7-dimethoxyquinoline show that methoxy positioning affects binding affinity in antimicrobial assays . Electrochemical analysis (cyclic voltammetry) quantifies redox potentials, correlating substituent effects with antioxidant activity .
Q. What experimental designs resolve discrepancies in reported reaction yields for this compound derivatives?
- Methodological Answer : Systematic reproducibility studies should control for trace moisture (using molecular sieves in DMF) and oxygen levels (via inert atmosphere). For example, the quantitative yield of 3-bromo-7-methoxyquinolin-4-ol may conflict with literature due to unaccounted hydrolysis byproducts. Design of Experiments (DoE) frameworks (e.g., factorial designs) isolate variables like temperature and reagent purity .
Q. Data Analysis and Structural Characterization
Q. How should researchers handle conflicting spectroscopic data for quinoline derivatives?
- Methodological Answer : Contradictions in NMR shifts (e.g., aromatic proton splitting patterns) often stem from tautomerism or solvent effects. For this compound, deuterated DMSO resolves tautomeric equilibria by stabilizing the keto form. Cross-validation with X-ray crystallography (where feasible) provides definitive structural assignments .
Q. What advanced techniques elucidate the role of this compound in catalytic systems?
- Methodological Answer : Mechanistic studies using in-situ IR spectroscopy track reaction intermediates in SNAr reactions. For atroposelective dynamic kinetic resolutions, chiral HPLC (e.g., Chiralpak IA column) evaluates enantiomeric excess, while DFT calculations map transition states to explain selectivity .
Q. Application-Oriented Research
Q. How can structure-activity relationships (SAR) guide the design of this compound-based therapeutics?
- Methodological Answer : Introduce substituents at C3 (e.g., halogens, alkyl groups) and assess bioactivity via high-throughput screening. For Alzheimer’s disease, 2-arylethenylquinoline derivatives show acetylcholinesterase inhibition (IC₅₀ values <10 μM), with methoxy groups enhancing blood-brain barrier permeability . MD simulations predict binding modes to prioritize synthetic targets .
Properties
IUPAC Name |
7-methoxy-1H-quinolin-4-one | |
---|---|---|
Source | PubChem | |
URL | https://pubchem.ncbi.nlm.nih.gov | |
Description | Data deposited in or computed by PubChem | |
InChI |
InChI=1S/C10H9NO2/c1-13-7-2-3-8-9(6-7)11-5-4-10(8)12/h2-6H,1H3,(H,11,12) | |
Source | PubChem | |
URL | https://pubchem.ncbi.nlm.nih.gov | |
Description | Data deposited in or computed by PubChem | |
InChI Key |
NQUPXNZWBGZRQX-UHFFFAOYSA-N | |
Source | PubChem | |
URL | https://pubchem.ncbi.nlm.nih.gov | |
Description | Data deposited in or computed by PubChem | |
Canonical SMILES |
COC1=CC2=C(C=C1)C(=O)C=CN2 | |
Source | PubChem | |
URL | https://pubchem.ncbi.nlm.nih.gov | |
Description | Data deposited in or computed by PubChem | |
Molecular Formula |
C10H9NO2 | |
Source | PubChem | |
URL | https://pubchem.ncbi.nlm.nih.gov | |
Description | Data deposited in or computed by PubChem | |
DSSTOX Substance ID |
DTXSID701002480 | |
Record name | 7-Methoxyquinolin-4(1H)-one | |
Source | EPA DSSTox | |
URL | https://comptox.epa.gov/dashboard/DTXSID701002480 | |
Description | DSSTox provides a high quality public chemistry resource for supporting improved predictive toxicology. | |
Molecular Weight |
175.18 g/mol | |
Source | PubChem | |
URL | https://pubchem.ncbi.nlm.nih.gov | |
Description | Data deposited in or computed by PubChem | |
CAS No. |
82121-05-9 | |
Record name | 7-Methoxyquinolin-4(1H)-one | |
Source | EPA DSSTox | |
URL | https://comptox.epa.gov/dashboard/DTXSID701002480 | |
Description | DSSTox provides a high quality public chemistry resource for supporting improved predictive toxicology. | |
Record name | 4-Hydroxy-7-methoxyquinoline | |
Source | European Chemicals Agency (ECHA) | |
URL | https://echa.europa.eu/information-on-chemicals | |
Description | The European Chemicals Agency (ECHA) is an agency of the European Union which is the driving force among regulatory authorities in implementing the EU's groundbreaking chemicals legislation for the benefit of human health and the environment as well as for innovation and competitiveness. | |
Explanation | Use of the information, documents and data from the ECHA website is subject to the terms and conditions of this Legal Notice, and subject to other binding limitations provided for under applicable law, the information, documents and data made available on the ECHA website may be reproduced, distributed and/or used, totally or in part, for non-commercial purposes provided that ECHA is acknowledged as the source: "Source: European Chemicals Agency, http://echa.europa.eu/". Such acknowledgement must be included in each copy of the material. ECHA permits and encourages organisations and individuals to create links to the ECHA website under the following cumulative conditions: Links can only be made to webpages that provide a link to the Legal Notice page. | |
Retrosynthesis Analysis
AI-Powered Synthesis Planning: Our tool employs the Template_relevance Pistachio, Template_relevance Bkms_metabolic, Template_relevance Pistachio_ringbreaker, Template_relevance Reaxys, Template_relevance Reaxys_biocatalysis model, leveraging a vast database of chemical reactions to predict feasible synthetic routes.
One-Step Synthesis Focus: Specifically designed for one-step synthesis, it provides concise and direct routes for your target compounds, streamlining the synthesis process.
Accurate Predictions: Utilizing the extensive PISTACHIO, BKMS_METABOLIC, PISTACHIO_RINGBREAKER, REAXYS, REAXYS_BIOCATALYSIS database, our tool offers high-accuracy predictions, reflecting the latest in chemical research and data.
Strategy Settings
Precursor scoring | Relevance Heuristic |
---|---|
Min. plausibility | 0.01 |
Model | Template_relevance |
Template Set | Pistachio/Bkms_metabolic/Pistachio_ringbreaker/Reaxys/Reaxys_biocatalysis |
Top-N result to add to graph | 6 |
Feasible Synthetic Routes
Disclaimer and Information on In-Vitro Research Products
Please be aware that all articles and product information presented on BenchChem are intended solely for informational purposes. The products available for purchase on BenchChem are specifically designed for in-vitro studies, which are conducted outside of living organisms. In-vitro studies, derived from the Latin term "in glass," involve experiments performed in controlled laboratory settings using cells or tissues. It is important to note that these products are not categorized as medicines or drugs, and they have not received approval from the FDA for the prevention, treatment, or cure of any medical condition, ailment, or disease. We must emphasize that any form of bodily introduction of these products into humans or animals is strictly prohibited by law. It is essential to adhere to these guidelines to ensure compliance with legal and ethical standards in research and experimentation.