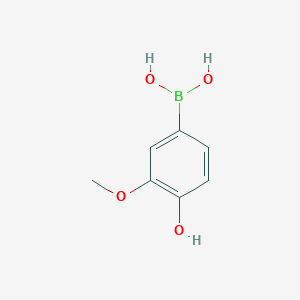
(4-Hydroxy-3-methoxyphenyl)boronic acid
Overview
Description
Mechanism of Action
Target of Action
(4-Hydroxy-3-methoxyphenyl)boronic acid, also known as 4-HYDROXY-3-METHOXYPHENYLBORONIC ACID, is primarily used as a reagent in the Suzuki–Miyaura cross-coupling reaction . This reaction is a widely-applied transition metal catalyzed carbon–carbon bond-forming reaction . The primary targets of this compound are the palladium complexes used in the Suzuki–Miyaura coupling .
Mode of Action
The Suzuki–Miyaura coupling reaction involves two key steps: oxidative addition and transmetalation . In the oxidative addition step, palladium becomes oxidized through its donation of electrons to form a new Pd–C bond . In the transmetalation step, the this compound, which is a nucleophilic organoboron reagent, is transferred from boron to palladium .
Biochemical Pathways
The Suzuki–Miyaura coupling reaction is a key biochemical pathway affected by this compound . This reaction is used to conjoin chemically differentiated fragments that participate in electronically divergent processes with the metal catalyst . The success of this reaction originates from a combination of exceptionally mild and functional group tolerant reaction conditions, with a relatively stable, readily prepared, and generally environmentally benign organoboron reagent .
Pharmacokinetics
It’s known that boronic acids, in general, are relatively stable and readily prepared . They are also generally environmentally benign, which suggests they may have good bioavailability .
Result of Action
The result of the action of this compound is the formation of new carbon–carbon bonds via the Suzuki–Miyaura coupling reaction . This reaction is widely used in organic synthesis, including the synthesis of pharmaceuticals, agrochemicals, and materials .
Action Environment
The action of this compound is influenced by several environmental factors. The Suzuki–Miyaura coupling reaction, in which this compound is used, requires mild and functional group tolerant reaction conditions . Additionally, the stability of the compound can be influenced by factors such as temperature and pH .
Biochemical Analysis
Biochemical Properties
4-Hydroxy-3-methoxyphenylboronic acid plays a significant role in biochemical reactions . It is known to interact with various enzymes, proteins, and other biomolecules .
Cellular Effects
It is believed to influence cell function, potentially impacting cell signaling pathways, gene expression, and cellular metabolism .
Molecular Mechanism
It is believed to exert its effects at the molecular level, potentially involving binding interactions with biomolecules, enzyme inhibition or activation, and changes in gene expression .
Temporal Effects in Laboratory Settings
Information on the product’s stability, degradation, and any long-term effects on cellular function observed in in vitro or in vivo studies is currently lacking .
Dosage Effects in Animal Models
The effects of 4-Hydroxy-3-methoxyphenylboronic acid vary with different dosages in animal models .
Transport and Distribution
Information on any transporters or binding proteins that it interacts with, as well as any effects on its localization or accumulation, is currently lacking .
Subcellular Localization
Information on any targeting signals or post-translational modifications that direct it to specific compartments or organelles is currently lacking .
Preparation Methods
Synthetic Routes and Reaction Conditions
The synthesis of (4-Hydroxy-3-methoxyphenyl)boronic acid typically involves the reaction of vanillin with boronic acid reagents. One common method is the Suzuki-Miyaura coupling reaction, which involves the palladium-catalyzed cross-coupling of an aryl halide with a boronic acid. The reaction conditions generally include a palladium catalyst, a base such as potassium carbonate, and a solvent like ethanol or water .
Industrial Production Methods
Industrial production of this compound may involve similar synthetic routes but on a larger scale. The process would be optimized for higher yields and cost-effectiveness, often using continuous flow reactors and automated systems to ensure consistent quality and efficiency.
Chemical Reactions Analysis
Types of Reactions
(4-Hydroxy-3-methoxyphenyl)boronic acid undergoes various types of chemical reactions, including:
Oxidation: It can be oxidized to form corresponding quinones.
Reduction: Reduction reactions can convert it into alcohol derivatives.
Substitution: It participates in nucleophilic substitution reactions, particularly in the presence of strong bases.
Common Reagents and Conditions
Oxidation: Common oxidizing agents include potassium permanganate and hydrogen peroxide.
Reduction: Reducing agents such as sodium borohydride or lithium aluminum hydride are often used.
Substitution: Strong bases like sodium hydroxide or potassium tert-butoxide facilitate substitution reactions.
Major Products Formed
The major products formed from these reactions depend on the specific conditions and reagents used. For example, oxidation may yield quinones, while reduction can produce alcohols. Substitution reactions can lead to various substituted phenylboronic acids.
Scientific Research Applications
(4-Hydroxy-3-methoxyphenyl)boronic acid has a wide range of applications in scientific research:
Comparison with Similar Compounds
Similar Compounds
3-Hydroxy-4-methoxyphenylboronic acid: Similar in structure but with different positioning of the hydroxyl and methoxy groups.
4-Hydroxyphenylboronic acid: Lacks the methoxy group, leading to different reactivity and applications.
3-Methoxyphenylboronic acid: Lacks the hydroxyl group, affecting its chemical properties and uses.
Uniqueness
(4-Hydroxy-3-methoxyphenyl)boronic acid is unique due to the presence of both hydroxyl and methoxy groups, which confer distinct reactivity and versatility in chemical synthesis. Its ability to participate in a wide range of reactions and form stable complexes with biomolecules makes it particularly valuable in research and industrial applications.
Properties
IUPAC Name |
(4-hydroxy-3-methoxyphenyl)boronic acid | |
---|---|---|
Source | PubChem | |
URL | https://pubchem.ncbi.nlm.nih.gov | |
Description | Data deposited in or computed by PubChem | |
InChI |
InChI=1S/C7H9BO4/c1-12-7-4-5(8(10)11)2-3-6(7)9/h2-4,9-11H,1H3 | |
Source | PubChem | |
URL | https://pubchem.ncbi.nlm.nih.gov | |
Description | Data deposited in or computed by PubChem | |
InChI Key |
UZFBWSFTHSYXCN-UHFFFAOYSA-N | |
Source | PubChem | |
URL | https://pubchem.ncbi.nlm.nih.gov | |
Description | Data deposited in or computed by PubChem | |
Canonical SMILES |
B(C1=CC(=C(C=C1)O)OC)(O)O | |
Source | PubChem | |
URL | https://pubchem.ncbi.nlm.nih.gov | |
Description | Data deposited in or computed by PubChem | |
Molecular Formula |
C7H9BO4 | |
Source | PubChem | |
URL | https://pubchem.ncbi.nlm.nih.gov | |
Description | Data deposited in or computed by PubChem | |
DSSTOX Substance ID |
DTXSID70468512 | |
Record name | (4-Hydroxy-3-methoxyphenyl)boronic acid | |
Source | EPA DSSTox | |
URL | https://comptox.epa.gov/dashboard/DTXSID70468512 | |
Description | DSSTox provides a high quality public chemistry resource for supporting improved predictive toxicology. | |
Molecular Weight |
167.96 g/mol | |
Source | PubChem | |
URL | https://pubchem.ncbi.nlm.nih.gov | |
Description | Data deposited in or computed by PubChem | |
CAS No. |
182344-21-4 | |
Record name | (4-Hydroxy-3-methoxyphenyl)boronic acid | |
Source | EPA DSSTox | |
URL | https://comptox.epa.gov/dashboard/DTXSID70468512 | |
Description | DSSTox provides a high quality public chemistry resource for supporting improved predictive toxicology. | |
Retrosynthesis Analysis
AI-Powered Synthesis Planning: Our tool employs the Template_relevance Pistachio, Template_relevance Bkms_metabolic, Template_relevance Pistachio_ringbreaker, Template_relevance Reaxys, Template_relevance Reaxys_biocatalysis model, leveraging a vast database of chemical reactions to predict feasible synthetic routes.
One-Step Synthesis Focus: Specifically designed for one-step synthesis, it provides concise and direct routes for your target compounds, streamlining the synthesis process.
Accurate Predictions: Utilizing the extensive PISTACHIO, BKMS_METABOLIC, PISTACHIO_RINGBREAKER, REAXYS, REAXYS_BIOCATALYSIS database, our tool offers high-accuracy predictions, reflecting the latest in chemical research and data.
Strategy Settings
Precursor scoring | Relevance Heuristic |
---|---|
Min. plausibility | 0.01 |
Model | Template_relevance |
Template Set | Pistachio/Bkms_metabolic/Pistachio_ringbreaker/Reaxys/Reaxys_biocatalysis |
Top-N result to add to graph | 6 |
Feasible Synthetic Routes
Q1: What is the significance of (4-Hydroxy-3-methoxyphenyl)boronic acid in the context of the presented research?
A1: this compound plays a crucial role as a reagent in the synthesis of eugenol. The research demonstrates the successful palladium-catalyzed allylic arylation of allyl phenyl ether with this compound pinacol ester to produce eugenol. [] This highlights the compound's utility in synthesizing complex molecules relevant to various fields, including fragrance and flavoring industries.
Disclaimer and Information on In-Vitro Research Products
Please be aware that all articles and product information presented on BenchChem are intended solely for informational purposes. The products available for purchase on BenchChem are specifically designed for in-vitro studies, which are conducted outside of living organisms. In-vitro studies, derived from the Latin term "in glass," involve experiments performed in controlled laboratory settings using cells or tissues. It is important to note that these products are not categorized as medicines or drugs, and they have not received approval from the FDA for the prevention, treatment, or cure of any medical condition, ailment, or disease. We must emphasize that any form of bodily introduction of these products into humans or animals is strictly prohibited by law. It is essential to adhere to these guidelines to ensure compliance with legal and ethical standards in research and experimentation.