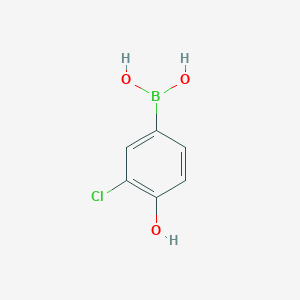
3-Chloro-4-hydroxyphenylboronic acid
Overview
Description
3-Chloro-4-hydroxyphenylboronic acid is a small molecule with the molecular formula C6H6BClO3. It has an average mass of 172.374 Da and a monoisotopic mass of 172.009857 Da . It is useful in crystal engineering studies due to the functional groups present .
Molecular Structure Analysis
The molecular structure of 3-Chloro-4-hydroxyphenylboronic acid consists of a benzene ring with a boronic acid group, a hydroxyl group, and a chlorine atom attached to it . The molecule is solid at 20°C .
Chemical Reactions Analysis
Boronic acids, including 3-Chloro-4-hydroxyphenylboronic acid, are known to participate in various chemical reactions. For instance, they are used in Suzuki-Miyaura cross-coupling reactions . They can also undergo protodeboronation .
Physical And Chemical Properties Analysis
3-Chloro-4-hydroxyphenylboronic acid has a density of 1.5±0.1 g/cm³, a boiling point of 360.6±52.0 °C at 760 mmHg, and a vapor pressure of 0.0±0.8 mmHg at 25°C . It also has an enthalpy of vaporization of 64.0±3.0 kJ/mol, a flash point of 171.9±30.7 °C, and an index of refraction of 1.602 .
Scientific Research Applications
Synthesis of Bio-supported Palladium Nanoparticles
The compound can be used in Suzuki-Miyaura coupling reactions . This reaction is used for the preparation of bio-supported palladium nanoparticles, which serve as phosphine-free catalysts .
Aminocarbonylation Reactions
3-Chloro-4-hydroxyphenylboronic acid can be used in palladium-catalyzed aminocarbonylation reactions . These reactions are crucial in the synthesis of amides from aryl halides .
Cross-Coupling Reactions
This compound is also used in cross-coupling reactions . These reactions are fundamental in the formation of carbon-carbon bonds, which are essential in organic synthesis .
Stille Coupling Reactions
Stille coupling reactions can also use 3-Chloro-4-hydroxyphenylboronic acid . These reactions are used to form carbon-carbon bonds between two different organic compounds .
Synthesis of Boron/Nitrogen-doped Polymer Nano/Microspheres
3-Chloro-4-hydroxyphenylboronic acid can be used to synthesize boron/nitrogen-doped polymer nano/microspheres . This is achieved through hydrothermal polymerization with formaldehyde and ammonia .
Formation of C-C Bond in the Presence of Pd Catalyst
This compound can be used in Suzuki-Miyaura coupling reactions with aryl halides for the formation of C-C bond in the presence of Pd catalyst .
Safety and Hazards
Mechanism of Action
Target of Action
3-Chloro-4-hydroxyphenylboronic acid is primarily used as a reagent in the Suzuki-Miyaura cross-coupling reactions . This reaction is a type of palladium-catalyzed carbon-carbon bond-forming process . The primary targets of this compound are the aryl halides or vinyl halides, with which it forms new carbon-carbon bonds .
Mode of Action
The compound interacts with its targets through a process called transmetalation . In this process, the organoboron compound (like 3-Chloro-4-hydroxyphenylboronic acid) transfers the organic group to a metal, in this case, palladium . This results in the formation of a new carbon-carbon bond, linking the two organic groups .
Biochemical Pathways
The Suzuki-Miyaura cross-coupling reaction, in which 3-Chloro-4-hydroxyphenylboronic acid participates, is a key step in various biochemical synthesis pathways . The new carbon-carbon bonds formed in this reaction can lead to the creation of complex organic compounds, including pharmaceuticals and polymers .
Pharmacokinetics
As a boronic acid, it is expected to have good stability and reactivity, making it suitable for use in various chemical reactions
Result of Action
The primary result of the action of 3-Chloro-4-hydroxyphenylboronic acid is the formation of new carbon-carbon bonds . This can lead to the synthesis of a wide range of organic compounds, including complex molecules used in pharmaceuticals and materials science .
Action Environment
The efficacy and stability of 3-Chloro-4-hydroxyphenylboronic acid can be influenced by various environmental factors. For instance, the Suzuki-Miyaura reaction requires a base and a palladium catalyst . The choice of these components, as well as the reaction temperature and solvent, can significantly affect the yield and selectivity of the reaction . Furthermore, the compound should be stored at room temperature and avoided from dust formation .
properties
IUPAC Name |
(3-chloro-4-hydroxyphenyl)boronic acid | |
---|---|---|
Source | PubChem | |
URL | https://pubchem.ncbi.nlm.nih.gov | |
Description | Data deposited in or computed by PubChem | |
InChI |
InChI=1S/C6H6BClO3/c8-5-3-4(7(10)11)1-2-6(5)9/h1-3,9-11H | |
Source | PubChem | |
URL | https://pubchem.ncbi.nlm.nih.gov | |
Description | Data deposited in or computed by PubChem | |
InChI Key |
WWQIKFZZILXJHG-UHFFFAOYSA-N | |
Source | PubChem | |
URL | https://pubchem.ncbi.nlm.nih.gov | |
Description | Data deposited in or computed by PubChem | |
Canonical SMILES |
B(C1=CC(=C(C=C1)O)Cl)(O)O | |
Source | PubChem | |
URL | https://pubchem.ncbi.nlm.nih.gov | |
Description | Data deposited in or computed by PubChem | |
Molecular Formula |
C6H6BClO3 | |
Source | PubChem | |
URL | https://pubchem.ncbi.nlm.nih.gov | |
Description | Data deposited in or computed by PubChem | |
DSSTOX Substance ID |
DTXSID50622630 | |
Record name | (3-Chloro-4-hydroxyphenyl)boronic acid | |
Source | EPA DSSTox | |
URL | https://comptox.epa.gov/dashboard/DTXSID50622630 | |
Description | DSSTox provides a high quality public chemistry resource for supporting improved predictive toxicology. | |
Molecular Weight |
172.37 g/mol | |
Source | PubChem | |
URL | https://pubchem.ncbi.nlm.nih.gov | |
Description | Data deposited in or computed by PubChem | |
Product Name |
3-Chloro-4-hydroxyphenylboronic acid | |
CAS RN |
182344-13-4 | |
Record name | (3-Chloro-4-hydroxyphenyl)boronic acid | |
Source | EPA DSSTox | |
URL | https://comptox.epa.gov/dashboard/DTXSID50622630 | |
Description | DSSTox provides a high quality public chemistry resource for supporting improved predictive toxicology. | |
Record name | 3-Chloro-4-hydroxyphenylboronic Acid | |
Source | European Chemicals Agency (ECHA) | |
URL | https://echa.europa.eu/information-on-chemicals | |
Description | The European Chemicals Agency (ECHA) is an agency of the European Union which is the driving force among regulatory authorities in implementing the EU's groundbreaking chemicals legislation for the benefit of human health and the environment as well as for innovation and competitiveness. | |
Explanation | Use of the information, documents and data from the ECHA website is subject to the terms and conditions of this Legal Notice, and subject to other binding limitations provided for under applicable law, the information, documents and data made available on the ECHA website may be reproduced, distributed and/or used, totally or in part, for non-commercial purposes provided that ECHA is acknowledged as the source: "Source: European Chemicals Agency, http://echa.europa.eu/". Such acknowledgement must be included in each copy of the material. ECHA permits and encourages organisations and individuals to create links to the ECHA website under the following cumulative conditions: Links can only be made to webpages that provide a link to the Legal Notice page. | |
Synthesis routes and methods I
Procedure details
Synthesis routes and methods II
Procedure details
Retrosynthesis Analysis
AI-Powered Synthesis Planning: Our tool employs the Template_relevance Pistachio, Template_relevance Bkms_metabolic, Template_relevance Pistachio_ringbreaker, Template_relevance Reaxys, Template_relevance Reaxys_biocatalysis model, leveraging a vast database of chemical reactions to predict feasible synthetic routes.
One-Step Synthesis Focus: Specifically designed for one-step synthesis, it provides concise and direct routes for your target compounds, streamlining the synthesis process.
Accurate Predictions: Utilizing the extensive PISTACHIO, BKMS_METABOLIC, PISTACHIO_RINGBREAKER, REAXYS, REAXYS_BIOCATALYSIS database, our tool offers high-accuracy predictions, reflecting the latest in chemical research and data.
Strategy Settings
Precursor scoring | Relevance Heuristic |
---|---|
Min. plausibility | 0.01 |
Model | Template_relevance |
Template Set | Pistachio/Bkms_metabolic/Pistachio_ringbreaker/Reaxys/Reaxys_biocatalysis |
Top-N result to add to graph | 6 |
Feasible Synthetic Routes
Disclaimer and Information on In-Vitro Research Products
Please be aware that all articles and product information presented on BenchChem are intended solely for informational purposes. The products available for purchase on BenchChem are specifically designed for in-vitro studies, which are conducted outside of living organisms. In-vitro studies, derived from the Latin term "in glass," involve experiments performed in controlled laboratory settings using cells or tissues. It is important to note that these products are not categorized as medicines or drugs, and they have not received approval from the FDA for the prevention, treatment, or cure of any medical condition, ailment, or disease. We must emphasize that any form of bodily introduction of these products into humans or animals is strictly prohibited by law. It is essential to adhere to these guidelines to ensure compliance with legal and ethical standards in research and experimentation.