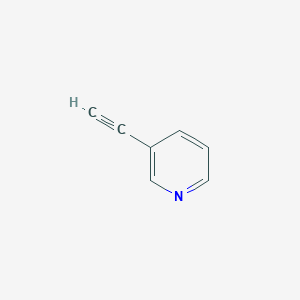
3-Ethynylpyridine
Overview
Description
3-Ethynylpyridine is a biochemical reagent . It is a compound with the molecular formula C7H5N . It contains an Alkyne group and can undergo copper-catalyzed azide-alkyne cycloaddition (CuAAc) with molecules containing Azide groups .
Synthesis Analysis
3-Ethynylpyridine is a click chemistry reagent . It contains an Alkyne group and can undergo copper-catalyzed azide-alkyne cycloaddition (CuAAc) with molecules containing Azide groups .
Molecular Structure Analysis
The molecular formula of 3-Ethynylpyridine is C7H5N . The molecular weight is 103.12 g/mol . The InChIKey of 3-Ethynylpyridine is CLRPXACRDTXENY-UHFFFAOYSA-N .
Chemical Reactions Analysis
3-Ethynylpyridine contains an Alkyne group and can undergo copper-catalyzed azide-alkyne cycloaddition (CuAAc) with molecules containing Azide groups .
Physical And Chemical Properties Analysis
The physical and chemical properties of 3-Ethynylpyridine include a molecular weight of 103.12 g/mol , a density of 1.0±0.1 g/cm3 , a boiling point of 170.5±13.0 °C at 760 mmHg , a vapour pressure of 1.9±0.3 mmHg at 25°C , an enthalpy of vaporization of 39.0±3.0 kJ/mol , a flash point of 57.5±12.4 °C , an index of refraction of 1.543 , a molar refractivity of 31.6±0.4 cm3 , a polar surface area of 13 Å2 , and a molar volume of 100.3±5.0 cm3 .
Scientific Research Applications
Molecular Electronics : The molecule 3-nitro-2-(3'-nitro-2'-ethynylpyridine)-5-thiopyridine, related to 3-ethynylpyridine, exhibits charge-induced conformational switching and rectifying behavior. It can be used in molecular electronics as a memory device operated by external fields or as a nano-actuator controlling molecular rotation (Derosa, Guda, & Seminario, 2003).
Organometallic Chemistry : Reactions involving 2-ethynylpyridine and iridium compounds lead to the formation of unique organometallic structures, demonstrating its role in the synthesis of novel compounds in organometallic chemistry (Le, Selnau, & Merola, 1994).
Material Science : 1,3-Dithiol-2-ylidene derivatives containing bis(ethynylpyridine) units exhibit unique crystal structures and charge-transfer interactions, highlighting their potential in the development of new materials (Kumagai, Tomura, Nishida, & Yamashita, 2003).
Agriculture : 2-Ethynylpyridine has been evaluated as a soil nitrification inhibitor, offering potential agricultural applications in managing nitrogen levels in soil (McCarty & Bremner, 1990).
Synthetic Chemistry : Ethynylpyridines can be synthesized through different methods and form charge-transfer complexes, indicating their utility in synthetic organic chemistry (Rodríguez, Martín-Villamil, Cano, & Fonseca, 1997).
Chemical Sensing : Ethynylpyridine derivatives can act as fluorescent chemosensors for metal ions like Zn2+, showcasing their applications in chemical sensing and environmental monitoring (Roy et al., 2016).
Mechanism of Action
Safety and Hazards
Future Directions
3-Ethynylpyridine is a biochemical reagent that can be used as a biological material or organic compound for life science related research . It is a click chemistry reagent, it contains an Alkyne group and can undergo copper-catalyzed azide-alkyne cycloaddition (CuAAc) with molecules containing Azide groups .
properties
IUPAC Name |
3-ethynylpyridine | |
---|---|---|
Source | PubChem | |
URL | https://pubchem.ncbi.nlm.nih.gov | |
Description | Data deposited in or computed by PubChem | |
InChI |
InChI=1S/C7H5N/c1-2-7-4-3-5-8-6-7/h1,3-6H | |
Source | PubChem | |
URL | https://pubchem.ncbi.nlm.nih.gov | |
Description | Data deposited in or computed by PubChem | |
InChI Key |
CLRPXACRDTXENY-UHFFFAOYSA-N | |
Source | PubChem | |
URL | https://pubchem.ncbi.nlm.nih.gov | |
Description | Data deposited in or computed by PubChem | |
Canonical SMILES |
C#CC1=CN=CC=C1 | |
Source | PubChem | |
URL | https://pubchem.ncbi.nlm.nih.gov | |
Description | Data deposited in or computed by PubChem | |
Molecular Formula |
C7H5N | |
Source | PubChem | |
URL | https://pubchem.ncbi.nlm.nih.gov | |
Description | Data deposited in or computed by PubChem | |
DSSTOX Substance ID |
DTXSID70947958 | |
Record name | 3-Ethynylpyridine | |
Source | EPA DSSTox | |
URL | https://comptox.epa.gov/dashboard/DTXSID70947958 | |
Description | DSSTox provides a high quality public chemistry resource for supporting improved predictive toxicology. | |
Molecular Weight |
103.12 g/mol | |
Source | PubChem | |
URL | https://pubchem.ncbi.nlm.nih.gov | |
Description | Data deposited in or computed by PubChem | |
Product Name |
3-Ethynylpyridine | |
CAS RN |
2510-23-8, 121697-66-3 | |
Record name | 3-Ethynylpyridine | |
Source | CAS Common Chemistry | |
URL | https://commonchemistry.cas.org/detail?cas_rn=2510-23-8 | |
Description | CAS Common Chemistry is an open community resource for accessing chemical information. Nearly 500,000 chemical substances from CAS REGISTRY cover areas of community interest, including common and frequently regulated chemicals, and those relevant to high school and undergraduate chemistry classes. This chemical information, curated by our expert scientists, is provided in alignment with our mission as a division of the American Chemical Society. | |
Explanation | The data from CAS Common Chemistry is provided under a CC-BY-NC 4.0 license, unless otherwise stated. | |
Record name | Pyridine, 3-ethynyl- | |
Source | ChemIDplus | |
URL | https://pubchem.ncbi.nlm.nih.gov/substance/?source=chemidplus&sourceid=0002510238 | |
Description | ChemIDplus is a free, web search system that provides access to the structure and nomenclature authority files used for the identification of chemical substances cited in National Library of Medicine (NLM) databases, including the TOXNET system. | |
Record name | 3-Ethynylpyridine | |
Source | EPA DSSTox | |
URL | https://comptox.epa.gov/dashboard/DTXSID70947958 | |
Description | DSSTox provides a high quality public chemistry resource for supporting improved predictive toxicology. | |
Record name | 3-Ethynylpyridine | |
Source | European Chemicals Agency (ECHA) | |
URL | https://echa.europa.eu/information-on-chemicals | |
Description | The European Chemicals Agency (ECHA) is an agency of the European Union which is the driving force among regulatory authorities in implementing the EU's groundbreaking chemicals legislation for the benefit of human health and the environment as well as for innovation and competitiveness. | |
Explanation | Use of the information, documents and data from the ECHA website is subject to the terms and conditions of this Legal Notice, and subject to other binding limitations provided for under applicable law, the information, documents and data made available on the ECHA website may be reproduced, distributed and/or used, totally or in part, for non-commercial purposes provided that ECHA is acknowledged as the source: "Source: European Chemicals Agency, http://echa.europa.eu/". Such acknowledgement must be included in each copy of the material. ECHA permits and encourages organisations and individuals to create links to the ECHA website under the following cumulative conditions: Links can only be made to webpages that provide a link to the Legal Notice page. | |
Record name | 3-Ethynylpyridine | |
Source | European Chemicals Agency (ECHA) | |
URL | https://echa.europa.eu/information-on-chemicals | |
Description | The European Chemicals Agency (ECHA) is an agency of the European Union which is the driving force among regulatory authorities in implementing the EU's groundbreaking chemicals legislation for the benefit of human health and the environment as well as for innovation and competitiveness. | |
Explanation | Use of the information, documents and data from the ECHA website is subject to the terms and conditions of this Legal Notice, and subject to other binding limitations provided for under applicable law, the information, documents and data made available on the ECHA website may be reproduced, distributed and/or used, totally or in part, for non-commercial purposes provided that ECHA is acknowledged as the source: "Source: European Chemicals Agency, http://echa.europa.eu/". Such acknowledgement must be included in each copy of the material. ECHA permits and encourages organisations and individuals to create links to the ECHA website under the following cumulative conditions: Links can only be made to webpages that provide a link to the Legal Notice page. | |
Retrosynthesis Analysis
AI-Powered Synthesis Planning: Our tool employs the Template_relevance Pistachio, Template_relevance Bkms_metabolic, Template_relevance Pistachio_ringbreaker, Template_relevance Reaxys, Template_relevance Reaxys_biocatalysis model, leveraging a vast database of chemical reactions to predict feasible synthetic routes.
One-Step Synthesis Focus: Specifically designed for one-step synthesis, it provides concise and direct routes for your target compounds, streamlining the synthesis process.
Accurate Predictions: Utilizing the extensive PISTACHIO, BKMS_METABOLIC, PISTACHIO_RINGBREAKER, REAXYS, REAXYS_BIOCATALYSIS database, our tool offers high-accuracy predictions, reflecting the latest in chemical research and data.
Strategy Settings
Precursor scoring | Relevance Heuristic |
---|---|
Min. plausibility | 0.01 |
Model | Template_relevance |
Template Set | Pistachio/Bkms_metabolic/Pistachio_ringbreaker/Reaxys/Reaxys_biocatalysis |
Top-N result to add to graph | 6 |
Feasible Synthetic Routes
Disclaimer and Information on In-Vitro Research Products
Please be aware that all articles and product information presented on BenchChem are intended solely for informational purposes. The products available for purchase on BenchChem are specifically designed for in-vitro studies, which are conducted outside of living organisms. In-vitro studies, derived from the Latin term "in glass," involve experiments performed in controlled laboratory settings using cells or tissues. It is important to note that these products are not categorized as medicines or drugs, and they have not received approval from the FDA for the prevention, treatment, or cure of any medical condition, ailment, or disease. We must emphasize that any form of bodily introduction of these products into humans or animals is strictly prohibited by law. It is essential to adhere to these guidelines to ensure compliance with legal and ethical standards in research and experimentation.