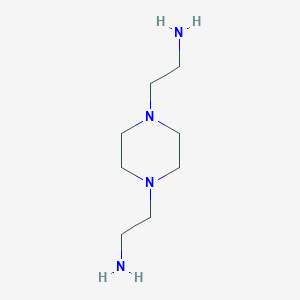
1,4-Piperazinediethanamine
Overview
Description
1,4-Piperazinediethanamine: is a chemical compound with the molecular formula C8H20N4 N,N’-Bis(2-aminoethyl)piperazine . This compound is a derivative of piperazine and is characterized by the presence of two aminoethyl groups attached to the piperazine ring. It is commonly used in various chemical and pharmaceutical applications due to its unique chemical properties .
Mechanism of Action
Mode of Action
It is likely that the compound interacts with its targets through its aminoethyl groups, but the exact nature of these interactions and the resulting changes are currently unknown .
Pharmacokinetics
Therefore, the impact of these properties on the bioavailability of the compound is currently unknown .
Biochemical Analysis
Biochemical Properties
Piperazine derivatives are known to interact with various enzymes and proteins, often acting as ligands or inhibitors . The nature of these interactions is largely dependent on the specific structure and functional groups of the piperazine derivative.
Cellular Effects
Other piperazine derivatives have been shown to influence cell function in various ways, such as modulating cell signaling pathways, altering gene expression, and affecting cellular metabolism .
Molecular Mechanism
It is known that piperazine derivatives can exert their effects at the molecular level through various mechanisms, including binding interactions with biomolecules, enzyme inhibition or activation, and changes in gene expression .
Preparation Methods
Synthetic Routes and Reaction Conditions: 1,4-Piperazinediethanamine can be synthesized through several methods. One common method involves the cyclization of 1,2-diamine derivatives with sulfonium salts. This reaction typically occurs under basic conditions and involves the formation of a piperazine ring . Another method includes the Ugi reaction, which is a multicomponent reaction that forms piperazine derivatives .
Industrial Production Methods: Industrial production of this compound often involves the reaction of piperazine with ethylene oxide or ethylene dichloride. These reactions are carried out under controlled conditions to ensure high yield and purity of the final product .
Chemical Reactions Analysis
Types of Reactions: 1,4-Piperazinediethanamine undergoes various chemical reactions, including:
Oxidation: This compound can be oxidized to form corresponding N-oxides.
Reduction: It can be reduced to form secondary amines.
Substitution: It can undergo nucleophilic substitution reactions to form various derivatives
Common Reagents and Conditions:
Oxidation: Common oxidizing agents include hydrogen peroxide and peracids.
Reduction: Reducing agents such as lithium aluminum hydride (LiAlH4) are often used.
Substitution: Nucleophiles like alkyl halides and acyl chlorides are commonly used in substitution reactions.
Major Products: The major products formed from these reactions include N-oxides, secondary amines, and various substituted derivatives .
Scientific Research Applications
1,4-Piperazinediethanamine has a wide range of applications in scientific research:
Comparison with Similar Compounds
Piperazine: A simpler analog with a six-membered ring containing two nitrogen atoms.
N,N’-Diethylpiperazine: A derivative with ethyl groups attached to the nitrogen atoms.
N,N’-Bis(2-aminoethyl)piperazine: Another derivative with aminoethyl groups attached to the nitrogen atoms
Uniqueness: 1,4-Piperazinediethanamine is unique due to its specific substitution pattern, which imparts distinct chemical and biological properties. Its ability to form stable complexes with various molecules makes it valuable in both research and industrial applications .
Properties
IUPAC Name |
2-[4-(2-aminoethyl)piperazin-1-yl]ethanamine | |
---|---|---|
Source | PubChem | |
URL | https://pubchem.ncbi.nlm.nih.gov | |
Description | Data deposited in or computed by PubChem | |
InChI |
InChI=1S/C8H20N4/c9-1-3-11-5-7-12(4-2-10)8-6-11/h1-10H2 | |
Source | PubChem | |
URL | https://pubchem.ncbi.nlm.nih.gov | |
Description | Data deposited in or computed by PubChem | |
InChI Key |
PAOXFRSJRCGJLV-UHFFFAOYSA-N | |
Source | PubChem | |
URL | https://pubchem.ncbi.nlm.nih.gov | |
Description | Data deposited in or computed by PubChem | |
Canonical SMILES |
C1CN(CCN1CCN)CCN | |
Source | PubChem | |
URL | https://pubchem.ncbi.nlm.nih.gov | |
Description | Data deposited in or computed by PubChem | |
Molecular Formula |
C8H20N4 | |
Source | PubChem | |
URL | https://pubchem.ncbi.nlm.nih.gov | |
Description | Data deposited in or computed by PubChem | |
DSSTOX Substance ID |
DTXSID6064400 | |
Record name | 1,4-Piperazinediethanamine | |
Source | EPA DSSTox | |
URL | https://comptox.epa.gov/dashboard/DTXSID6064400 | |
Description | DSSTox provides a high quality public chemistry resource for supporting improved predictive toxicology. | |
Molecular Weight |
172.27 g/mol | |
Source | PubChem | |
URL | https://pubchem.ncbi.nlm.nih.gov | |
Description | Data deposited in or computed by PubChem | |
CAS No. |
6531-38-0 | |
Record name | 1,4-Piperazinediethanamine | |
Source | CAS Common Chemistry | |
URL | https://commonchemistry.cas.org/detail?cas_rn=6531-38-0 | |
Description | CAS Common Chemistry is an open community resource for accessing chemical information. Nearly 500,000 chemical substances from CAS REGISTRY cover areas of community interest, including common and frequently regulated chemicals, and those relevant to high school and undergraduate chemistry classes. This chemical information, curated by our expert scientists, is provided in alignment with our mission as a division of the American Chemical Society. | |
Explanation | The data from CAS Common Chemistry is provided under a CC-BY-NC 4.0 license, unless otherwise stated. | |
Record name | 1,4-Piperazinediethylamine | |
Source | ChemIDplus | |
URL | https://pubchem.ncbi.nlm.nih.gov/substance/?source=chemidplus&sourceid=0006531380 | |
Description | ChemIDplus is a free, web search system that provides access to the structure and nomenclature authority files used for the identification of chemical substances cited in National Library of Medicine (NLM) databases, including the TOXNET system. | |
Record name | 1,4-Piperazinediethanamine | |
Source | EPA Chemicals under the TSCA | |
URL | https://www.epa.gov/chemicals-under-tsca | |
Description | EPA Chemicals under the Toxic Substances Control Act (TSCA) collection contains information on chemicals and their regulations under TSCA, including non-confidential content from the TSCA Chemical Substance Inventory and Chemical Data Reporting. | |
Record name | 1,4-Piperazinediethanamine | |
Source | EPA DSSTox | |
URL | https://comptox.epa.gov/dashboard/DTXSID6064400 | |
Description | DSSTox provides a high quality public chemistry resource for supporting improved predictive toxicology. | |
Record name | Piperazine-1,4-diethylamine | |
Source | European Chemicals Agency (ECHA) | |
URL | https://echa.europa.eu/substance-information/-/substanceinfo/100.026.754 | |
Description | The European Chemicals Agency (ECHA) is an agency of the European Union which is the driving force among regulatory authorities in implementing the EU's groundbreaking chemicals legislation for the benefit of human health and the environment as well as for innovation and competitiveness. | |
Explanation | Use of the information, documents and data from the ECHA website is subject to the terms and conditions of this Legal Notice, and subject to other binding limitations provided for under applicable law, the information, documents and data made available on the ECHA website may be reproduced, distributed and/or used, totally or in part, for non-commercial purposes provided that ECHA is acknowledged as the source: "Source: European Chemicals Agency, http://echa.europa.eu/". Such acknowledgement must be included in each copy of the material. ECHA permits and encourages organisations and individuals to create links to the ECHA website under the following cumulative conditions: Links can only be made to webpages that provide a link to the Legal Notice page. | |
Record name | 1,4-PIPERAZINEDIETHYLAMINE | |
Source | FDA Global Substance Registration System (GSRS) | |
URL | https://gsrs.ncats.nih.gov/ginas/app/beta/substances/4H2W4524XV | |
Description | The FDA Global Substance Registration System (GSRS) enables the efficient and accurate exchange of information on what substances are in regulated products. Instead of relying on names, which vary across regulatory domains, countries, and regions, the GSRS knowledge base makes it possible for substances to be defined by standardized, scientific descriptions. | |
Explanation | Unless otherwise noted, the contents of the FDA website (www.fda.gov), both text and graphics, are not copyrighted. They are in the public domain and may be republished, reprinted and otherwise used freely by anyone without the need to obtain permission from FDA. Credit to the U.S. Food and Drug Administration as the source is appreciated but not required. | |
Retrosynthesis Analysis
AI-Powered Synthesis Planning: Our tool employs the Template_relevance Pistachio, Template_relevance Bkms_metabolic, Template_relevance Pistachio_ringbreaker, Template_relevance Reaxys, Template_relevance Reaxys_biocatalysis model, leveraging a vast database of chemical reactions to predict feasible synthetic routes.
One-Step Synthesis Focus: Specifically designed for one-step synthesis, it provides concise and direct routes for your target compounds, streamlining the synthesis process.
Accurate Predictions: Utilizing the extensive PISTACHIO, BKMS_METABOLIC, PISTACHIO_RINGBREAKER, REAXYS, REAXYS_BIOCATALYSIS database, our tool offers high-accuracy predictions, reflecting the latest in chemical research and data.
Strategy Settings
Precursor scoring | Relevance Heuristic |
---|---|
Min. plausibility | 0.01 |
Model | Template_relevance |
Template Set | Pistachio/Bkms_metabolic/Pistachio_ringbreaker/Reaxys/Reaxys_biocatalysis |
Top-N result to add to graph | 6 |
Feasible Synthetic Routes
Disclaimer and Information on In-Vitro Research Products
Please be aware that all articles and product information presented on BenchChem are intended solely for informational purposes. The products available for purchase on BenchChem are specifically designed for in-vitro studies, which are conducted outside of living organisms. In-vitro studies, derived from the Latin term "in glass," involve experiments performed in controlled laboratory settings using cells or tissues. It is important to note that these products are not categorized as medicines or drugs, and they have not received approval from the FDA for the prevention, treatment, or cure of any medical condition, ailment, or disease. We must emphasize that any form of bodily introduction of these products into humans or animals is strictly prohibited by law. It is essential to adhere to these guidelines to ensure compliance with legal and ethical standards in research and experimentation.