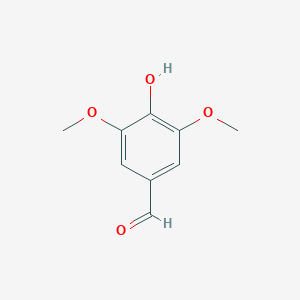
Syringaldehyde
Overview
Description
Syringaldehyde, also known as 4-hydroxy-3,5-dimethoxybenzaldehyde, is an organic compound that occurs naturally in trace amounts. It is a colorless solid that is soluble in alcohol and polar organic solvents. This compound is found in the wood of spruce and maple trees and is also formed in oak barrels, contributing to the flavor profile of whisky . This compound is used by some insect species in their chemical communication systems .
Mechanism of Action
Target of Action
Syringaldehyde, a phenolic aldehyde, has been found to interact with several targets. It has shown promising anticancer activity against a range of women-specific cancer cell lines, including A2780 (ovarian cancer), HeLa and C33a (cervical cancer), and MDA-MB-453, MDA-MB-231 and MCF-7 (breast cancer) . It also inhibits the type III secretion system (T3SS) of Salmonella enterica , and has been shown to have an antihyperglycemic effect in diabetic rats by increasing glucose utilization and insulin sensitivity .
Mode of Action
This compound’s mode of action varies depending on the target. In cancer cells, it induces cell apoptosis and G2/M phase arrest by causing significant DNA damage and provoking a strong DNA damage response . It also affects the phosphorylation and distribution of FAK, thereby inhibiting cell adhesion and migration . In Salmonella enterica, this compound inhibits the expression of important effector proteins (SipA, SipB and SipC) at a concentration of 0.18 mM without affecting bacterial growth .
Biochemical Pathways
This compound is derived from lignin, a complex organic polymer that is abundant in nature . It is produced through the oxidation of lignin, which yields aromatic aldehydes such as this compound . The biochemical pathways affected by this compound are largely dependent on its targets and mode of action.
Pharmacokinetics
It has been shown to have an antihyperglycemic effect in diabetic rats, suggesting that it is bioavailable and can exert systemic effects . In cancer studies, tissue distribution experiments indicated that a compound derived from this compound tended to accumulate in the ovary in vivo .
Result of Action
The results of this compound’s action are diverse and depend on the target. In cancer cells, it induces apoptosis and cell cycle arrest, leading to decreased proliferation . In Salmonella enterica, it inhibits bacterial invasion and cellular injury . In diabetic rats, it increases glucose utilization and insulin sensitivity, leading to an antihyperglycemic effect .
Preparation Methods
Syringaldehyde can be synthesized through several methods:
Duff Reaction: This method involves the reaction of syringol with hexamethylenetetramine in the presence of an acid catalyst.
Catalytic Oxidation of Lignin: Using perovskite-type oxides as catalysts, this compound can be prepared from lignin.
Condensation of 2,6-Dimethoxyphenol with Chloroform: This method, although less efficient, involves the condensation of 2,6-dimethoxyphenol with chloroform in an alkaline solution.
Chemical Reactions Analysis
Scientific Research Applications
Syringaldehyde has diverse applications in scientific research:
Comparison with Similar Compounds
Properties
IUPAC Name |
4-hydroxy-3,5-dimethoxybenzaldehyde | |
---|---|---|
Source | PubChem | |
URL | https://pubchem.ncbi.nlm.nih.gov | |
Description | Data deposited in or computed by PubChem | |
InChI |
InChI=1S/C9H10O4/c1-12-7-3-6(5-10)4-8(13-2)9(7)11/h3-5,11H,1-2H3 | |
Source | PubChem | |
URL | https://pubchem.ncbi.nlm.nih.gov | |
Description | Data deposited in or computed by PubChem | |
InChI Key |
KCDXJAYRVLXPFO-UHFFFAOYSA-N | |
Source | PubChem | |
URL | https://pubchem.ncbi.nlm.nih.gov | |
Description | Data deposited in or computed by PubChem | |
Canonical SMILES |
COC1=CC(=CC(=C1O)OC)C=O | |
Source | PubChem | |
URL | https://pubchem.ncbi.nlm.nih.gov | |
Description | Data deposited in or computed by PubChem | |
Molecular Formula |
C9H10O4 | |
Source | PubChem | |
URL | https://pubchem.ncbi.nlm.nih.gov | |
Description | Data deposited in or computed by PubChem | |
DSSTOX Substance ID |
DTXSID2059643 | |
Record name | Syringaldehyde | |
Source | EPA DSSTox | |
URL | https://comptox.epa.gov/dashboard/DTXSID2059643 | |
Description | DSSTox provides a high quality public chemistry resource for supporting improved predictive toxicology. | |
Molecular Weight |
182.17 g/mol | |
Source | PubChem | |
URL | https://pubchem.ncbi.nlm.nih.gov | |
Description | Data deposited in or computed by PubChem | |
Physical Description |
Pale yellow solid; [Merck Index], Very pale green needles; Alcoholic aroma | |
Record name | Syringaldehyde | |
Source | Haz-Map, Information on Hazardous Chemicals and Occupational Diseases | |
URL | https://haz-map.com/Agents/11526 | |
Description | Haz-Map® is an occupational health database designed for health and safety professionals and for consumers seeking information about the adverse effects of workplace exposures to chemical and biological agents. | |
Explanation | Copyright (c) 2022 Haz-Map(R). All rights reserved. Unless otherwise indicated, all materials from Haz-Map are copyrighted by Haz-Map(R). No part of these materials, either text or image may be used for any purpose other than for personal use. Therefore, reproduction, modification, storage in a retrieval system or retransmission, in any form or by any means, electronic, mechanical or otherwise, for reasons other than personal use, is strictly prohibited without prior written permission. | |
Record name | 4-Hydroxy-3,5-dimethoxy benzaldehyde | |
Source | Joint FAO/WHO Expert Committee on Food Additives (JECFA) | |
URL | https://www.fao.org/food/food-safety-quality/scientific-advice/jecfa/jecfa-flav/details/en/c/1856/ | |
Description | The flavoring agent databse provides the most recent specifications for flavorings evaluated by JECFA. | |
Explanation | Permission from WHO is not required for the use of WHO materials issued under the Creative Commons Attribution-NonCommercial-ShareAlike 3.0 Intergovernmental Organization (CC BY-NC-SA 3.0 IGO) licence. | |
Solubility |
Insoluble in water, Soluble (in ethanol) | |
Record name | 4-Hydroxy-3,5-dimethoxy benzaldehyde | |
Source | Joint FAO/WHO Expert Committee on Food Additives (JECFA) | |
URL | https://www.fao.org/food/food-safety-quality/scientific-advice/jecfa/jecfa-flav/details/en/c/1856/ | |
Description | The flavoring agent databse provides the most recent specifications for flavorings evaluated by JECFA. | |
Explanation | Permission from WHO is not required for the use of WHO materials issued under the Creative Commons Attribution-NonCommercial-ShareAlike 3.0 Intergovernmental Organization (CC BY-NC-SA 3.0 IGO) licence. | |
CAS No. |
134-96-3 | |
Record name | Syringaldehyde | |
Source | CAS Common Chemistry | |
URL | https://commonchemistry.cas.org/detail?cas_rn=134-96-3 | |
Description | CAS Common Chemistry is an open community resource for accessing chemical information. Nearly 500,000 chemical substances from CAS REGISTRY cover areas of community interest, including common and frequently regulated chemicals, and those relevant to high school and undergraduate chemistry classes. This chemical information, curated by our expert scientists, is provided in alignment with our mission as a division of the American Chemical Society. | |
Explanation | The data from CAS Common Chemistry is provided under a CC-BY-NC 4.0 license, unless otherwise stated. | |
Record name | Syringaldehyde | |
Source | ChemIDplus | |
URL | https://pubchem.ncbi.nlm.nih.gov/substance/?source=chemidplus&sourceid=0000134963 | |
Description | ChemIDplus is a free, web search system that provides access to the structure and nomenclature authority files used for the identification of chemical substances cited in National Library of Medicine (NLM) databases, including the TOXNET system. | |
Record name | SYRINGALDEHYDE | |
Source | DTP/NCI | |
URL | https://dtp.cancer.gov/dtpstandard/servlet/dwindex?searchtype=NSC&outputformat=html&searchlist=41153 | |
Description | The NCI Development Therapeutics Program (DTP) provides services and resources to the academic and private-sector research communities worldwide to facilitate the discovery and development of new cancer therapeutic agents. | |
Explanation | Unless otherwise indicated, all text within NCI products is free of copyright and may be reused without our permission. Credit the National Cancer Institute as the source. | |
Record name | Benzaldehyde, 4-hydroxy-3,5-dimethoxy- | |
Source | EPA Chemicals under the TSCA | |
URL | https://www.epa.gov/chemicals-under-tsca | |
Description | EPA Chemicals under the Toxic Substances Control Act (TSCA) collection contains information on chemicals and their regulations under TSCA, including non-confidential content from the TSCA Chemical Substance Inventory and Chemical Data Reporting. | |
Record name | Syringaldehyde | |
Source | EPA DSSTox | |
URL | https://comptox.epa.gov/dashboard/DTXSID2059643 | |
Description | DSSTox provides a high quality public chemistry resource for supporting improved predictive toxicology. | |
Record name | 4-hydroxy-3,5-dimethoxybenzaldehyde | |
Source | European Chemicals Agency (ECHA) | |
URL | https://echa.europa.eu/substance-information/-/substanceinfo/100.004.698 | |
Description | The European Chemicals Agency (ECHA) is an agency of the European Union which is the driving force among regulatory authorities in implementing the EU's groundbreaking chemicals legislation for the benefit of human health and the environment as well as for innovation and competitiveness. | |
Explanation | Use of the information, documents and data from the ECHA website is subject to the terms and conditions of this Legal Notice, and subject to other binding limitations provided for under applicable law, the information, documents and data made available on the ECHA website may be reproduced, distributed and/or used, totally or in part, for non-commercial purposes provided that ECHA is acknowledged as the source: "Source: European Chemicals Agency, http://echa.europa.eu/". Such acknowledgement must be included in each copy of the material. ECHA permits and encourages organisations and individuals to create links to the ECHA website under the following cumulative conditions: Links can only be made to webpages that provide a link to the Legal Notice page. | |
Record name | SYRINGALDEHYDE | |
Source | FDA Global Substance Registration System (GSRS) | |
URL | https://gsrs.ncats.nih.gov/ginas/app/beta/substances/2ZR01KTT21 | |
Description | The FDA Global Substance Registration System (GSRS) enables the efficient and accurate exchange of information on what substances are in regulated products. Instead of relying on names, which vary across regulatory domains, countries, and regions, the GSRS knowledge base makes it possible for substances to be defined by standardized, scientific descriptions. | |
Explanation | Unless otherwise noted, the contents of the FDA website (www.fda.gov), both text and graphics, are not copyrighted. They are in the public domain and may be republished, reprinted and otherwise used freely by anyone without the need to obtain permission from FDA. Credit to the U.S. Food and Drug Administration as the source is appreciated but not required. | |
Synthesis routes and methods I
Procedure details
Synthesis routes and methods II
Procedure details
Synthesis routes and methods III
Procedure details
Retrosynthesis Analysis
AI-Powered Synthesis Planning: Our tool employs the Template_relevance Pistachio, Template_relevance Bkms_metabolic, Template_relevance Pistachio_ringbreaker, Template_relevance Reaxys, Template_relevance Reaxys_biocatalysis model, leveraging a vast database of chemical reactions to predict feasible synthetic routes.
One-Step Synthesis Focus: Specifically designed for one-step synthesis, it provides concise and direct routes for your target compounds, streamlining the synthesis process.
Accurate Predictions: Utilizing the extensive PISTACHIO, BKMS_METABOLIC, PISTACHIO_RINGBREAKER, REAXYS, REAXYS_BIOCATALYSIS database, our tool offers high-accuracy predictions, reflecting the latest in chemical research and data.
Strategy Settings
Precursor scoring | Relevance Heuristic |
---|---|
Min. plausibility | 0.01 |
Model | Template_relevance |
Template Set | Pistachio/Bkms_metabolic/Pistachio_ringbreaker/Reaxys/Reaxys_biocatalysis |
Top-N result to add to graph | 6 |
Feasible Synthetic Routes
Disclaimer and Information on In-Vitro Research Products
Please be aware that all articles and product information presented on BenchChem are intended solely for informational purposes. The products available for purchase on BenchChem are specifically designed for in-vitro studies, which are conducted outside of living organisms. In-vitro studies, derived from the Latin term "in glass," involve experiments performed in controlled laboratory settings using cells or tissues. It is important to note that these products are not categorized as medicines or drugs, and they have not received approval from the FDA for the prevention, treatment, or cure of any medical condition, ailment, or disease. We must emphasize that any form of bodily introduction of these products into humans or animals is strictly prohibited by law. It is essential to adhere to these guidelines to ensure compliance with legal and ethical standards in research and experimentation.