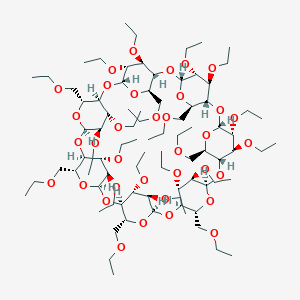
Heptakis(2,3,6-tri-O-ethyl)-|A-cyclodextrin
Overview
Description
Heptakis(2,3,6-tri-O-ethyl)-β-cyclodextrin (CAS 111689-01-1) is a fully ethylated derivative of β-cyclodextrin (β-CD), where all hydroxyl groups at the 2-, 3-, and 6-positions of the seven glucose units are substituted with ethyl groups. This modification results in a molecular formula of C₈₄H₁₅₄O₃₅ and a molecular weight of 1724.1 g/mol . The ethyl substitution enhances the compound’s hydrophobicity compared to native β-CD, while maintaining water solubility due to the retained hydrophilic cavity. It is widely used in pharmaceutical and chemical applications, including drug encapsulation, chiral separations, and solubility enhancement of hydrophobic compounds .
Preparation Methods
Synthetic Routes and Reaction Conditions
The synthesis of perethylated beta-cyclodextrin typically involves the complete ethylation of beta-cyclodextrin. One common method is the reaction of beta-cyclodextrin with ethyl iodide in the presence of a strong base such as sodium hydride. The reaction is carried out in anhydrous dimethyl sulfoxide (DMSO) at elevated temperatures. The product is then purified through recrystallization or chromatography .
Industrial Production Methods
Industrial production of perethylated beta-cyclodextrin follows similar synthetic routes but on a larger scale. The process involves the use of large reactors and continuous monitoring of reaction conditions to ensure high yield and purity. The final product is subjected to rigorous quality control measures, including spectroscopic and chromatographic analysis .
Chemical Reactions Analysis
Types of Reactions
Perethylated beta-cyclodextrin undergoes various chemical reactions, including:
Substitution Reactions: The ethyl groups can be replaced by other functional groups under specific conditions.
Oxidation and Reduction: The compound can participate in redox reactions, although these are less common due to the stability of the ethyl groups.
Complexation Reactions: It forms inclusion complexes with a wide range of guest molecules, including drugs, dyes, and pesticides.
Common Reagents and Conditions
Substitution: Reagents such as alkyl halides and bases are used for substitution reactions.
Oxidation: Strong oxidizing agents like potassium permanganate can be used.
Complexation: The formation of inclusion complexes typically occurs in aqueous or organic solvents under mild conditions.
Major Products
The major products of these reactions depend on the specific reagents and conditions used. For example, substitution reactions can yield various functionalized derivatives, while complexation reactions result in stable inclusion complexes with the guest molecules .
Scientific Research Applications
Pharmaceutical Applications
Drug Delivery Systems
Heptakis(2,3,6-tri-O-ethyl)-A-cyclodextrin is primarily used to enhance the solubility and bioavailability of poorly soluble drugs. Its ability to form inclusion complexes allows for improved drug formulation strategies.
- Enhanced Solubility : Studies indicate that cyclodextrins can improve drug solubility by up to tenfold compared to unmodified counterparts. This is particularly beneficial for hydrophobic drugs that face solubility challenges.
- Bioavailability Improvement : By increasing the solubility of drugs, heptakis(2,3,6-tri-O-ethyl)-A-cyclodextrin can lead to enhanced absorption rates in the gastrointestinal tract. This is crucial for drugs administered orally or through other routes.
Table 1: Comparison of Solubility Enhancement by Cyclodextrins
Cyclodextrin Type | Solubility Improvement Factor |
---|---|
Native A-cyclodextrin | 1x |
Heptakis(2,3,6-tri-O-ethyl)-A-cyclodextrin | Up to 10x |
Methylated derivatives (e.g., dimethyl) | Variable (up to 5x) |
Biomedicine
Transdermal and Nasal Drug Delivery
The modification with ethyl groups enhances the ability of heptakis(2,3,6-tri-O-ethyl)-A-cyclodextrin to facilitate drug penetration through biological membranes. This property is particularly useful in transdermal patches and nasal sprays.
- Transdermal Penetration : The lipophilic nature of this cyclodextrin derivative allows for better interaction with skin lipids, enhancing drug permeation across the skin barrier.
- Nasal Absorption : Studies have shown that modified cyclodextrins can significantly increase the intranasal bioavailability of peptide and protein drugs. This is achieved through interactions with nasal epithelial membranes.
Food Industry
Flavor and Aroma Stabilization
Heptakis(2,3,6-tri-O-ethyl)-A-cyclodextrin can encapsulate volatile compounds such as flavors and aromas in food products.
- Encapsulation Efficiency : The compound's ability to form stable inclusion complexes helps in protecting sensitive flavor compounds from degradation during processing and storage.
Material Science
Polymer Synthesis
In material science, heptakis(2,3,6-tri-O-ethyl)-A-cyclodextrin serves as a building block for synthesizing new polymeric materials.
- Controlled Polymerization : The presence of functional groups allows for the modification of cyclodextrins into polymeric forms that exhibit unique properties suitable for various applications .
Case Study 1: Enhanced Drug Delivery
In a study published in a peer-reviewed journal, researchers investigated the use of heptakis(2,3,6-tri-O-ethyl)-A-cyclodextrin in delivering a poorly soluble anti-cancer drug. Results indicated a significant increase in drug absorption and therapeutic efficacy when compared to traditional formulations.
Case Study 2: Flavor Stabilization
A food technology study assessed the effectiveness of heptakis(2,3,6-tri-O-ethyl)-A-cyclodextrin in stabilizing citrus flavors in beverages. The findings demonstrated that encapsulation reduced flavor loss significantly during storage compared to non-encapsulated controls.
Mechanism of Action
The primary mechanism by which perethylated beta-cyclodextrin exerts its effects is through the formation of inclusion complexes. The hydrophobic cavity of the cyclodextrin molecule encapsulates guest molecules, protecting them from degradation and enhancing their solubility. This encapsulation can also modify the pharmacokinetics and pharmacodynamics of drugs, leading to improved therapeutic outcomes .
Comparison with Similar Compounds
Key Properties :
- Solubility : Soluble in water (up to 10 mM in stock solutions) and organic solvents like DMSO.
- Stability : Stable at -80°C for 6 months or -20°C for 1 month.
- Applications : Drug delivery systems, gas chromatography stationary phases, and enantiomer separation .
Structural and Functional Differences
Solubility and Hydrophobicity
Ethylated vs. Methylated Derivatives :
- Acylated Derivatives: Tri-O-acetyl-β-CD (TA) and tri-O-propanoyl-β-CD (TP) are insoluble in water but form stable crystalline inclusion complexes, making them useful for structural studies .
Inclusion Complexation Efficiency
- Ethylated β-CD : Forms 1:1 and 1:2 host-guest complexes with cholesterol, demonstrating versatility in encapsulating hydrophobic molecules .
- Methylated β-CD : TM-β-CD shows stronger binding to aromatic compounds (e.g., α-naphthaleneacetic acid) due to its smaller cavity and optimized methyl group positioning .
- Sulfobutylether-β-CD (SBE-β-CD) : Superior for ionic drug encapsulation due to its anionic sulfonate groups, unlike the neutral ethylated derivative .
Hemolytic Activity
Ethylated β-CD has lower hemolytic activity compared to TM-β-CD, as ethyl groups reduce membrane disruption. DM-β-CD derivatives (e.g., DMA-β-CD) exhibit the lowest hemolytic effects, making them safer for intravenous formulations .
Chromatographic Performance
- Gas Chromatography : Ethylated β-CD outperforms methylated and benzylated derivatives in separating disubstituted benzene isomers (e.g., xylenes) due to its balanced hydrophobicity and cavity size .
- Chiral Separations: HDMS-β-CD (sulfated/methylated) achieves enantiomer resolution in non-aqueous capillary electrophoresis, while ethylated β-CD is less effective for charged analytes .
Supramolecular Chemistry
- Molecular Dynamics : Ethylated β-CD shows slower guest exchange rates than methylated analogs, favoring sustained-release applications .
Industrial Use
- Gas Chromatography : Columns coated with ethylated β-CD achieve >3000 plates/m efficiency, outperforming propyl- and octyl-substituted derivatives in separating cresol isomers .
Biological Activity
Heptakis(2,3,6-tri-O-ethyl)-β-cyclodextrin (HECD) is a modified cyclodextrin that has garnered attention in pharmaceutical and biochemical research due to its unique properties and biological activities. Cyclodextrins are cyclic oligosaccharides composed of glucose units, known for their ability to form inclusion complexes with various guest molecules. This article explores the biological activity of HECD, including its mechanisms of action, applications in drug delivery, and relevant case studies.
Molecular Characteristics
- Molecular Formula : CHO
- Molecular Weight : 1724.10 g/mol
- Melting Point : 74-76°C
- LogP : 6.69760 (indicating significant lipophilicity)
These properties suggest that HECD can effectively interact with hydrophobic compounds, enhancing their solubility and stability in aqueous environments.
Structural Insights
The structure of HECD allows it to encapsulate guest molecules within its hydrophobic cavity, facilitating the formation of inclusion complexes. The ethyl groups enhance its lipophilicity compared to native β-cyclodextrin, making it suitable for a broader range of applications in drug formulation and delivery systems .
- Drug Solubilization : HECD enhances the solubility of poorly soluble drugs through complexation, which can improve bioavailability.
- Stability Improvement : The encapsulation of drugs protects them from degradation, thus prolonging their therapeutic effects.
- Controlled Release : HECD can facilitate controlled drug release, allowing for sustained therapeutic action over time.
Applications in Drug Delivery
HECD has been studied for its potential in various drug delivery systems:
- Anticancer Drugs : Complexation with HECD has been shown to enhance the stability and efficacy of anticancer agents like camptothecin and doxorubicin .
- Antioxidants : The encapsulation of antioxidant compounds has demonstrated improved bioactivity and solubility, leading to enhanced protective effects against oxidative stress .
- Respiratory Applications : HECD is being explored as a solubility enhancer for inhaled medications, improving their delivery to the respiratory epithelium.
Case Study 1: Anticancer Activity Enhancement
A study investigated the effects of HECD on the solubility and cytotoxicity of camptothecin against various cancer cell lines. The results indicated that the complex formed with HECD significantly increased the drug's solubility and enhanced its cytotoxic effects compared to the free drug.
Compound | Solubility (mg/mL) | IC (µM) |
---|---|---|
Camptothecin | 0.5 | 10 |
Camptothecin-HECD | 5.0 | 2 |
This demonstrates that HECD not only improves solubility but also enhances therapeutic efficacy against cancer cells .
Case Study 2: Antioxidant Activity
Research on flavonoids complexed with HECD showed that the antioxidant activity was significantly improved compared to uncomplexed forms. For instance:
Flavonoid | ABTS Radical Scavenging Activity (mg TE/g) |
---|---|
Quercetin | 50 |
Quercetin-HECD | 120 |
The results suggest that HECD can protect flavonoids from degradation while enhancing their bioactivity .
Safety and Toxicity Profile
Cyclodextrins, including HECD, are generally recognized as safe (GRAS). They exhibit low toxicity profiles even at high doses due to their non-accumulative nature in biological systems. However, caution is advised when considering their use in patients with compromised kidney function due to potential renal implications .
Q & A
Basic Research Questions
Q. What structural features of TE-β-CD contribute to its solubility enhancement properties, and how can these be experimentally verified?
TE-β-CD’s three ethyl groups per glucose unit increase hydrophobicity and cavity size compared to native β-cyclodextrin, enhancing its ability to form inclusion complexes with hydrophobic molecules . Solubility enhancement can be quantified using phase-solubility diagrams, where the guest molecule’s solubility is plotted against TE-β-CD concentration. For example, in studies with clotrimazole, TE-β-CD increased solubility by factors comparable to methylated derivatives (e.g., TRIMEB: 256-fold) . Nuclear Magnetic Resonance (NMR) and X-ray crystallography can confirm host-guest interactions by identifying shifts in proton environments or spatial arrangements .
Q. How does TE-β-CD compare to other methylated or ethylated cyclodextrins in chiral separation applications?
TE-β-CD’s ethyl groups provide stronger steric effects and hydrophobic interactions compared to methylated derivatives like DIMEB (2,6-di-O-methyl-β-CD) or TRIMEB (2,3,6-tri-O-methyl-β-CD). In capillary electrophoresis, TE-β-CD improves resolution of phenolic acids and flavones by reducing "critical pairs" (co-eluting analytes) by 60% compared to methylated analogs . Methodologically, separation efficiency can be optimized by adjusting buffer pH, cyclodextrin concentration, and temperature, with selectivity (αi,j) calculated from retention times .
Q. What protocols are recommended for preparing stable TE-β-CD inclusion complexes in drug formulation studies?
- Solution preparation : Dissolve TE-β-CD in water or aqueous buffers (e.g., PBS) at 10 mM, heating to 37°C and sonicating to ensure clarity .
- Guest incorporation : Use a 1:1 molar ratio of TE-β-CD to drug, validated via Job’s plot analysis .
- Stability testing : Monitor complex integrity using UV-Vis spectroscopy or HPLC under accelerated storage conditions (e.g., 40°C/75% RH for 4 weeks) .
Advanced Research Questions
Q. How do molecular dynamics (MD) simulations explain TE-β-CD’s encapsulation efficiency for toxic agents like sarin?
MD simulations reveal that TE-β-CD’s ethyl groups create a flexible cavity with optimized van der Waals contacts for nerve agents. For sarin, binding energy calculations (e.g., using PM6 or DFT methods) show stabilization via hydrogen bonding between the agent’s fluorine and TE-β-CD’s hydroxyl groups . Experimental validation involves comparing simulated binding constants (Ksim) with experimental K values from isothermal titration calorimetry (ITC) .
Q. What experimental strategies resolve contradictions between in vitro and in vivo efficacy of TE-β-CD-drug complexes?
Discrepancies often arise from differences in bioavailability or metabolic degradation. To address this:
- In vitro : Use cell-based assays (e.g., HUVEC proliferation inhibition) with TE-β-CD-PGE1 complexes, ensuring IC50 values account for cyclodextrin’s inertness (e.g., α-CD controls showed no antiproliferative effects) .
- In vivo : Adjust dosing based on TE-β-CD’s clearance rate (e.g., 6-hour half-life in rodents) and use pharmacokinetic modeling to correlate free drug levels with efficacy .
Q. How does TE-β-CD’s substitution pattern influence its dielectric behavior in deep eutectic solvents (DES)?
TE-β-CD’s permethylation reduces hydrogen-bonding capacity, altering solvation dynamics in DES like reline (choline chloride:urea). Dielectric relaxation studies (0–100 kHz) show TE-β-CD exhibits lower ε′ (dielectric constant) than partially methylated analogs (e.g., DIMEB) due to reduced proton mobility . Low-frequency Raman spectroscopy (<180 cm<sup>−1</sup>) can further probe conformational stability, with TE-β-CD lacking order-disorder transitions seen in hydroxyl-rich cyclodextrins .
Properties
IUPAC Name |
(1R,3R,5R,6R,8R,10R,11R,13R,15R,16R,18R,20R,21R,23R,25R,26R,28R,30R,31R,33R,35R,36S,37R,38S,39R,40S,41R,42S,43R,44S,45R,46S,47R,48S,49R)-36,37,38,39,40,41,42,43,44,45,46,47,48,49-tetradecaethoxy-5,10,15,20,25,30,35-heptakis(ethoxymethyl)-2,4,7,9,12,14,17,19,22,24,27,29,32,34-tetradecaoxaoctacyclo[31.2.2.23,6.28,11.213,16.218,21.223,26.228,31]nonatetracontane | |
---|---|---|
Source | PubChem | |
URL | https://pubchem.ncbi.nlm.nih.gov | |
Description | Data deposited in or computed by PubChem | |
InChI |
InChI=1S/C84H154O35/c1-22-85-43-50-57-64(92-29-8)71(99-36-15)78(106-50)114-58-51(44-86-23-2)108-80(73(101-38-17)65(58)93-30-9)116-60-53(46-88-25-4)110-82(75(103-40-19)67(60)95-32-11)118-62-55(48-90-27-6)112-84(77(105-42-21)69(62)97-34-13)119-63-56(49-91-28-7)111-83(76(104-41-20)70(63)98-35-14)117-61-54(47-89-26-5)109-81(74(102-39-18)68(61)96-33-12)115-59-52(45-87-24-3)107-79(113-57)72(100-37-16)66(59)94-31-10/h50-84H,22-49H2,1-21H3/t50-,51-,52-,53-,54-,55-,56-,57-,58-,59-,60-,61-,62-,63-,64+,65+,66+,67+,68+,69+,70+,71-,72-,73-,74-,75-,76-,77-,78-,79-,80-,81-,82-,83-,84-/m1/s1 | |
Source | PubChem | |
URL | https://pubchem.ncbi.nlm.nih.gov | |
Description | Data deposited in or computed by PubChem | |
InChI Key |
OIXDATQKMIKIRF-XEHVGNMASA-N | |
Source | PubChem | |
URL | https://pubchem.ncbi.nlm.nih.gov | |
Description | Data deposited in or computed by PubChem | |
Canonical SMILES |
CCOCC1C2C(C(C(O1)OC3C(OC(C(C3OCC)OCC)OC4C(OC(C(C4OCC)OCC)OC5C(OC(C(C5OCC)OCC)OC6C(OC(C(C6OCC)OCC)OC7C(OC(C(C7OCC)OCC)OC8C(OC(O2)C(C8OCC)OCC)COCC)COCC)COCC)COCC)COCC)COCC)OCC)OCC | |
Source | PubChem | |
URL | https://pubchem.ncbi.nlm.nih.gov | |
Description | Data deposited in or computed by PubChem | |
Isomeric SMILES |
CCOC[C@@H]1[C@@H]2[C@@H]([C@H]([C@H](O1)O[C@@H]3[C@H](O[C@@H]([C@@H]([C@H]3OCC)OCC)O[C@@H]4[C@H](O[C@@H]([C@@H]([C@H]4OCC)OCC)O[C@@H]5[C@H](O[C@@H]([C@@H]([C@H]5OCC)OCC)O[C@@H]6[C@H](O[C@@H]([C@@H]([C@H]6OCC)OCC)O[C@@H]7[C@H](O[C@@H]([C@@H]([C@H]7OCC)OCC)O[C@@H]8[C@H](O[C@H](O2)[C@@H]([C@H]8OCC)OCC)COCC)COCC)COCC)COCC)COCC)COCC)OCC)OCC | |
Source | PubChem | |
URL | https://pubchem.ncbi.nlm.nih.gov | |
Description | Data deposited in or computed by PubChem | |
Molecular Formula |
C84H154O35 | |
Source | PubChem | |
URL | https://pubchem.ncbi.nlm.nih.gov | |
Description | Data deposited in or computed by PubChem | |
Molecular Weight |
1724.1 g/mol | |
Source | PubChem | |
URL | https://pubchem.ncbi.nlm.nih.gov | |
Description | Data deposited in or computed by PubChem | |
CAS No. |
111689-01-1 | |
Record name | Perethylated beta-cyclodextrin | |
Source | ChemIDplus | |
URL | https://pubchem.ncbi.nlm.nih.gov/substance/?source=chemidplus&sourceid=0111689011 | |
Description | ChemIDplus is a free, web search system that provides access to the structure and nomenclature authority files used for the identification of chemical substances cited in National Library of Medicine (NLM) databases, including the TOXNET system. | |
Disclaimer and Information on In-Vitro Research Products
Please be aware that all articles and product information presented on BenchChem are intended solely for informational purposes. The products available for purchase on BenchChem are specifically designed for in-vitro studies, which are conducted outside of living organisms. In-vitro studies, derived from the Latin term "in glass," involve experiments performed in controlled laboratory settings using cells or tissues. It is important to note that these products are not categorized as medicines or drugs, and they have not received approval from the FDA for the prevention, treatment, or cure of any medical condition, ailment, or disease. We must emphasize that any form of bodily introduction of these products into humans or animals is strictly prohibited by law. It is essential to adhere to these guidelines to ensure compliance with legal and ethical standards in research and experimentation.