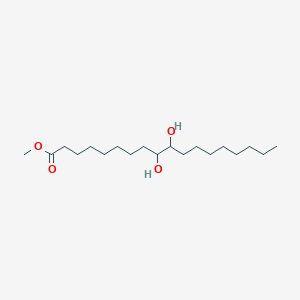
Methyl 9,10-dihydroxyoctadecanoate
Overview
Description
Methyl 9,10-dihydroxyoctadecanoate (CAS 1115-01-1) is a dihydroxy fatty acid methyl ester derived from the hydroxylation of unsaturated methyl esters found in seed oils, such as Blighia unijugata . Its molecular formula is C₁₉H₃₈O₄, with a molecular weight of 330.50 g/mol .
Preparation Methods
Catalytic Hydroxylation-Esterification of Oleic Acid
The most common route to methyl 9,10-dihydroxyoctadecanoate begins with oleic acid (cis-9-octadecenoic acid), which undergoes hydroxylation at the double bond followed by esterification with methanol.
Phosphotungstic Acid-Catalyzed Hydroxylation
A patent by CN112812001A describes a one-pot method using phosphotungstic acid (H₃PW₁₂O₄₀) or its supported variants as catalysts. Oleic acid is mixed with the catalyst, and hydrogen peroxide (30–50 wt%) is added dropwise at 20–80°C. The reaction completes in 0.5–24 hours, after which the aqueous layer is separated, and the organic layer is washed and dried.
Key Advantages :
-
Eliminates sulfuric or formic acid, reducing equipment corrosion .
-
Catalyst recyclability up to five cycles without significant activity loss .
Limitations :
-
Requires precise control of H₂O₂ addition to prevent over-oxidation.
Permanganate-Mediated Hydroxylation with Subsequent Esterification
Traditional methods employ potassium permanganate (KMnO₄) under alkaline conditions for hydroxylation, followed by esterification.
Hydroxylation with KMnO₄
In a procedure detailed by ScienceAsia , oleic acid is dissolved in 1% NaOH and cooled to 0–10°C. A 1% KMnO₄ solution is added slowly, and the mixture is stirred until the purple color dissipates. Excess permanganate is reduced with Na₂SO₃, and the product is acidified to precipitate 9,10-dihydroxystearic acid (yield: 47%) .
Esterification Using Montmorillonite KSF
The dihydroxy acid is esterified with methanol using montmorillonite KSF, a solid acid catalyst, under ultrasound irradiation (42 kHz, 10 minutes). This method achieves an 88% yield of this compound .
Reaction Conditions :
Parameter | Value |
---|---|
Catalyst Loading | 20 wt% relative to substrate |
Temperature | Room temperature |
Sonication Duration | 10 minutes |
Advantages :
Comparative Analysis of Esterification Methods
Esterification efficiency varies significantly with catalysts and techniques. The table below contrasts conventional and sonochemical approaches:
Method | Catalyst | Time (min) | Yield (%) | Reference |
---|---|---|---|---|
Reflux | H₂SO₄ | 180 | 72 | |
Sonication | Montmorillonite | 10 | 94 | |
Magnetic Stirring | Amberlyst-15 | 120 | 85 |
Sonication enhances mass transfer and catalyst-substrate interaction, enabling near-quantitative yields . Heterogeneous catalysts like montmorillonite KSF outperform homogeneous acids (e.g., H₂SO₄) by simplifying product isolation and reducing waste .
Green Synthesis via Acetalization-Esterification Tandem Reactions
Emerging strategies combine acetalization and esterification to streamline production. For example, 9,10-dihydroxystearic acid is first converted to a dioxolane intermediate using n-pentanal, which is then esterified with methanol. This tandem process, catalyzed by p-toluenesulfonic acid (p-TSA), achieves an 82% overall yield while avoiding harsh oxidants .
Mechanistic Insights :
-
Acetalization : The diol reacts with n-pentanal to form a cyclic dioxolane, protecting the hydroxyl groups .
-
Esterification : The protected acid reacts with methanol under mild conditions, followed by acidic workup to remove the acetal .
Industrial-Scale Considerations
Catalyst Selection
Supported phosphotungstic acid (e.g., on SiO₂ or TiO₂) is preferred for fixed-bed reactors due to its mechanical stability and high surface area .
Solvent-Free Systems
Recent patents emphasize solvent-free conditions to minimize waste. For instance, oleic acid and methanol are reacted in a molar ratio of 1:5 with 2% H₃PW₁₂O₄₀/SiO₂ at 70°C, achieving 91% conversion in 4 hours .
Environmental Impact
Life-cycle assessments highlight that sonochemical methods reduce energy consumption by 40% compared to conventional heating . Additionally, phosphotungstic acid catalysts generate no toxic byproducts, aligning with REACH regulations .
Chemical Reactions Analysis
Types of Reactions: Methyl 9,10-dihydroxystearate undergoes various chemical reactions, including:
Oxidation: The compound can be further oxidized to produce carboxylic acids.
Reduction: Reduction reactions can convert it back to its precursor forms.
Substitution: It can participate in substitution reactions to form different derivatives.
Common Reagents and Conditions:
Oxidation: Hydrogen peroxide (H₂O₂) and catalysts like phosphotungstic acid.
Reduction: Reducing agents such as sodium borohydride (NaBH₄).
Substitution: Various nucleophiles under acidic or basic conditions.
Major Products:
Oxidation: Carboxylic acids such as azelaic acid and pelargonic acid.
Reduction: Methyl oleate and other reduced forms.
Substitution: Various substituted esters and derivatives
Scientific Research Applications
Scientific Research Applications
1. Chemistry
- Surfactants and Lubricants : Methyl 9,10-dihydroxyoctadecanoate serves as an intermediate in the synthesis of surfactants and lubricants, enhancing their performance due to its hydroxyl groups that improve solubility and surface activity .
2. Biology
- Biological Activities : Research indicates that this compound exhibits potential biological activities, including antioxidant properties and interactions with biological membranes. It is being studied for its role in lipid metabolism and potential therapeutic effects .
3. Medicine
- Therapeutic Properties : Investigations into the compound's therapeutic properties suggest possible applications in pharmaceuticals, particularly in formulations aimed at treating conditions related to oxidative stress and inflammation .
4. Material Science
- Biodegradable Materials : The compound's structure allows it to be used in developing bio-based lubricants and coatings, contributing to sustainable materials science .
Industrial Applications
1. Cosmetic Industry
- Skin Care Formulations : Due to its moisturizing properties, this compound is explored as an ingredient in skin care products, offering benefits such as hydration and improved skin barrier function .
2. Polymer Production
- Ester Lubricants : It is utilized in producing ester lubricants that are biodegradable and derived from renewable resources, aligning with environmental sustainability goals .
Case Study 1: Surfactant Development
A study focused on synthesizing surfactants from this compound demonstrated that these surfactants exhibited superior emulsifying properties compared to traditional surfactants. The research highlighted the compound's ability to stabilize oil-in-water emulsions effectively.
Case Study 2: Antioxidant Activity
Research investigating the antioxidant activity of this compound found that it significantly reduced oxidative stress markers in cellular models. This suggests potential applications in nutraceutical formulations aimed at combating oxidative damage.
Data Table: Summary of Applications
Application Area | Specific Use | Benefits |
---|---|---|
Chemistry | Intermediate for surfactants | Enhanced solubility and surface activity |
Biology | Study of biological interactions | Insights into lipid metabolism |
Medicine | Potential therapeutic agent | Anti-inflammatory and antioxidant properties |
Cosmetic Industry | Ingredient in skin care products | Improved hydration and skin barrier function |
Material Science | Production of biodegradable lubricants | Sustainable alternatives to traditional materials |
Mechanism of Action
The mechanism of action of methyl 9,10-dihydroxystearate involves its interaction with various molecular targets. In the presence of hydrogen peroxide and a tungsten-based catalyst, the compound undergoes dihydroxylation, forming peroxo-phosphotungstate anions. These anions facilitate the dihydroxylation of methyl oleate, leading to the formation of methyl 9,10-dihydroxystearate. The recyclability of the catalyst and the optimal concentration of hydrogen peroxide are crucial for the efficiency of this reaction .
Comparison with Similar Compounds
Physical Properties
Key properties include:
Structural and Functional Differences
Methyl 9,10-dihydroxyoctadecanoate is distinguished by two vicinal hydroxyl groups at C9 and C10. Below is a comparative analysis with structurally related compounds:
Key Observations
Polarity and Reactivity: The vicinal diol structure enhances polarity, increasing solubility in polar solvents and enabling diverse chemical modifications (e.g., esterification, ketalization) compared to mono-hydroxy or epoxy analogs .
Thermal Behavior: Heating this compound at 290–300°C yields 9(10)-oxooctadecanoate, whereas epoxy analogs undergo ring-opening reactions .
Stereochemical Specificity : The (9S,10S) stereoisomer exhibits biological relevance in insect metabolism, highlighting the importance of configuration in bioactivity .
Green Synthesis: CTAP-mediated synthesis avoids solvents, contrasting with methods requiring harsh reagents (e.g., KMnO₄ in acidic/basic conditions) .
Application Differences
- Bio-lubricants: this compound-derived esters exhibit superior lubricant properties (e.g., low iodine value, high oxidation stability) compared to epoxy or mono-hydroxy analogs .
- Biological Roles: The (9S,10S) stereoisomer is a silk gland metabolite, while mono-hydroxy variants are linked to lipid oxidation pathways .
Biological Activity
Methyl 9,10-dihydroxyoctadecanoate (MDHO), a diol compound derived from octadecanoic acid, has garnered significant attention in recent years due to its diverse biological activities and potential applications in various fields, including biochemistry and material science. This article delves into the biological activity of MDHO, highlighting its antioxidant properties, interactions with biological molecules, and implications for health and industry.
This compound has the molecular formula C₁₉H₃₈O₄ and features two hydroxyl groups located at the 9th and 10th carbon positions of an octadecanoate chain. This unique positioning contributes to its reactivity and biological properties compared to other fatty acids.
Biological Activities
1. Antioxidant Properties
MDHO exhibits notable antioxidant activity, which is primarily attributed to its hydroxyl groups. These functional groups can donate hydrogen atoms to free radicals, thereby neutralizing them and reducing oxidative stress in biological systems. Studies have demonstrated that MDHO can protect cellular components from oxidative damage, suggesting potential applications in nutraceutical formulations aimed at enhancing health and preventing diseases related to oxidative stress.
2. Interaction with Biological Molecules
Research indicates that MDHO interacts synergistically with other bioactive compounds and fatty acids. These interactions may enhance its therapeutic effects, making it a candidate for developing effective formulations in pharmaceuticals and nutraceuticals. Understanding these interactions is crucial for optimizing its use in various applications .
3. Potential Health Benefits
MDHO's biological activity extends to potential health benefits, including anti-inflammatory effects. Preliminary studies suggest that it may modulate inflammatory pathways, which could be beneficial in managing chronic inflammatory conditions. Further research is necessary to elucidate these mechanisms and confirm its efficacy in clinical settings .
Table 1: Summary of Biological Activities of this compound
Case Study: Antioxidant Activity Assessment
In a study assessing the antioxidant capacity of MDHO, researchers employed various assays such as DPPH radical scavenging and ABTS radical cation decolorization methods. The results indicated that MDHO exhibited significant scavenging activity comparable to known antioxidants like ascorbic acid. This finding supports the compound's potential use in dietary supplements aimed at reducing oxidative stress.
Q & A
Basic Research Questions
Q. What are the standard protocols for synthesizing methyl 9,10-dihydroxyoctadecanoate, and how are stereoisomers controlled during synthesis?
this compound is synthesized via microbial hydration of methyl oleate or ricinoleate, followed by stereospecific hydroxylation. For example, methyl threo-9,10-dihydroxyoctadecanoate (CAS 3639-31-4) and methyl erythro-9,10-dihydroxyoctadecanoate (CAS 3639-30-3) are stereoisomers derived from oleic acid and ricinoleic acid, respectively . Reaction conditions (e.g., microbial strain selection, pH, temperature) critically influence stereochemical outcomes. NMR and chiral chromatography are used to verify stereopurity .
Q. Which analytical techniques are most effective for characterizing this compound and its derivatives?
Key methods include:
- NMR Spectroscopy : - and -NMR for hydroxyl group positioning (e.g., δ 3.67 ppm for methoxy groups in -NMR) .
- Mass Spectrometry (MS) : Electron ionization (EI-MS) and silylation (e.g., di-OTMS derivatives) for fragmentation pattern analysis, distinguishing positional isomers like 9,10- vs. 10,12-dihydroxy derivatives .
- FTIR : Identification of ester carbonyl (1740–1720 cm) and hydroxyl (3400–3200 cm) functional groups .
Q. What are the key physical properties (e.g., solubility, stability) relevant to handling this compound in laboratory settings?
The compound is a viscous liquid with a density of 0.968 g/cm, boiling point of 443.1°C, and low water solubility. Storage requires anhydrous conditions to prevent hydrolysis. Stability tests under varying pH and temperature (e.g., 25–60°C) are recommended for long-term studies .
Advanced Research Questions
Q. How does this compound serve as a precursor for bio-lubricants, and what reaction pathways optimize its performance?
The compound is esterified with dicarboxylic acids (e.g., oxalic or malonic acid) using p-toluenesulfonic acid (p-TSA) as a catalyst to form cyclic dioxane/dioxepane derivatives. These derivatives exhibit improved lubricant properties:
- Oxalic acid derivative : 93.9% yield, iodine value (IV) < 5 g I/100g, total acid number (TAN) < 0.5 mg KOH/g.
- Malonic acid derivative : 89.6% yield, enhanced oxidative stability due to reduced unsaturation .
Q. What enzymatic pathways involve this compound, and how are its metabolites regulated?
The compound is a substrate for bifunctional epoxide hydrolase 2 (EC 3.1.3.76), which catalyzes its dephosphorylation to (9S,10S)-9,10-dihydroxyoctadecanoate in lipid metabolism. This enzyme is implicated in peroxisomal pathways and arachidonic acid metabolism, with kinetic parameters (e.g., , ) critical for modeling metabolic flux .
Q. How do micellar structures of this compound derivatives influence their surfactant properties?
The compound’s gemini surfactant derivatives form micelles with aggregation numbers dependent on stereochemistry. For example, threo-isomers exhibit lower critical micelle concentrations (CMC) than erythro-isomers due to optimized hydrophobic packing. Small-angle X-ray scattering (SAXS) and dynamic light scattering (DLS) are used to characterize micelle size and morphology .
Q. Data Contradictions and Methodological Challenges
Q. Why do conflicting reports exist regarding the oxidative stability of this compound-based lubricants?
Discrepancies arise from variations in:
- Catalyst type : Homogeneous (p-TSA) vs. heterogeneous catalysts (e.g., zeolites) affect side reactions and purity.
- Accelerated aging protocols : ASTM D2272 (150°C, 72 hr) vs. modified conditions (e.g., 100°C, 168 hr) yield different oxidation rates .
Q. How can researchers resolve ambiguities in mass spectral fragmentation patterns of dihydroxy derivatives?
Comparative analyses with isotopically labeled standards (e.g., - or -labeled analogs) and tandem MS (MS/MS) improve peak assignment accuracy. For example, allylic fragmentation pathways dominate in unsaturated derivatives, while saturated analogs show simpler patterns .
Q. Methodological Recommendations
Q. What protocols ensure reproducibility in synthesizing stereoisomerically pure this compound?
- Use enantiomerically pure starting materials (e.g., methyl R-ricinoleate).
- Employ chiral stationary phases (e.g., Chiralpak AD-H) for HPLC purification.
- Validate purity via optical rotation () and NOESY NMR .
Q. How should researchers design experiments to assess the compound’s role in lipid signaling pathways?
Properties
IUPAC Name |
methyl 9,10-dihydroxyoctadecanoate | |
---|---|---|
Source | PubChem | |
URL | https://pubchem.ncbi.nlm.nih.gov | |
Description | Data deposited in or computed by PubChem | |
InChI |
InChI=1S/C19H38O4/c1-3-4-5-6-8-11-14-17(20)18(21)15-12-9-7-10-13-16-19(22)23-2/h17-18,20-21H,3-16H2,1-2H3 | |
Source | PubChem | |
URL | https://pubchem.ncbi.nlm.nih.gov | |
Description | Data deposited in or computed by PubChem | |
InChI Key |
RITHLQKJQSKUAO-UHFFFAOYSA-N | |
Source | PubChem | |
URL | https://pubchem.ncbi.nlm.nih.gov | |
Description | Data deposited in or computed by PubChem | |
Canonical SMILES |
CCCCCCCCC(C(CCCCCCCC(=O)OC)O)O | |
Source | PubChem | |
URL | https://pubchem.ncbi.nlm.nih.gov | |
Description | Data deposited in or computed by PubChem | |
Molecular Formula |
C19H38O4 | |
Source | PubChem | |
URL | https://pubchem.ncbi.nlm.nih.gov | |
Description | Data deposited in or computed by PubChem | |
DSSTOX Substance ID |
DTXSID10880895 | |
Record name | octadecanoic acid, 9,10-dihydroxy-, methyl ester | |
Source | EPA DSSTox | |
URL | https://comptox.epa.gov/dashboard/DTXSID10880895 | |
Description | DSSTox provides a high quality public chemistry resource for supporting improved predictive toxicology. | |
Molecular Weight |
330.5 g/mol | |
Source | PubChem | |
URL | https://pubchem.ncbi.nlm.nih.gov | |
Description | Data deposited in or computed by PubChem | |
CAS No. |
1115-01-1 | |
Record name | Methyl 9,10-dihydroxystearate | |
Source | CAS Common Chemistry | |
URL | https://commonchemistry.cas.org/detail?cas_rn=1115-01-1 | |
Description | CAS Common Chemistry is an open community resource for accessing chemical information. Nearly 500,000 chemical substances from CAS REGISTRY cover areas of community interest, including common and frequently regulated chemicals, and those relevant to high school and undergraduate chemistry classes. This chemical information, curated by our expert scientists, is provided in alignment with our mission as a division of the American Chemical Society. | |
Explanation | The data from CAS Common Chemistry is provided under a CC-BY-NC 4.0 license, unless otherwise stated. | |
Record name | Methyl 9,10-dihydroxyoctadecanoate | |
Source | ChemIDplus | |
URL | https://pubchem.ncbi.nlm.nih.gov/substance/?source=chemidplus&sourceid=0001115011 | |
Description | ChemIDplus is a free, web search system that provides access to the structure and nomenclature authority files used for the identification of chemical substances cited in National Library of Medicine (NLM) databases, including the TOXNET system. | |
Record name | Methyl 9,10-dihydroxystearate | |
Source | DTP/NCI | |
URL | https://dtp.cancer.gov/dtpstandard/servlet/dwindex?searchtype=NSC&outputformat=html&searchlist=409398 | |
Description | The NCI Development Therapeutics Program (DTP) provides services and resources to the academic and private-sector research communities worldwide to facilitate the discovery and development of new cancer therapeutic agents. | |
Explanation | Unless otherwise indicated, all text within NCI products is free of copyright and may be reused without our permission. Credit the National Cancer Institute as the source. | |
Record name | octadecanoic acid, 9,10-dihydroxy-, methyl ester | |
Source | EPA DSSTox | |
URL | https://comptox.epa.gov/dashboard/DTXSID10880895 | |
Description | DSSTox provides a high quality public chemistry resource for supporting improved predictive toxicology. | |
Record name | Methyl 9,10-dihydroxyoctadecanoate | |
Source | European Chemicals Agency (ECHA) | |
URL | https://echa.europa.eu/substance-information/-/substanceinfo/100.012.928 | |
Description | The European Chemicals Agency (ECHA) is an agency of the European Union which is the driving force among regulatory authorities in implementing the EU's groundbreaking chemicals legislation for the benefit of human health and the environment as well as for innovation and competitiveness. | |
Explanation | Use of the information, documents and data from the ECHA website is subject to the terms and conditions of this Legal Notice, and subject to other binding limitations provided for under applicable law, the information, documents and data made available on the ECHA website may be reproduced, distributed and/or used, totally or in part, for non-commercial purposes provided that ECHA is acknowledged as the source: "Source: European Chemicals Agency, http://echa.europa.eu/". Such acknowledgement must be included in each copy of the material. ECHA permits and encourages organisations and individuals to create links to the ECHA website under the following cumulative conditions: Links can only be made to webpages that provide a link to the Legal Notice page. | |
Retrosynthesis Analysis
AI-Powered Synthesis Planning: Our tool employs the Template_relevance Pistachio, Template_relevance Bkms_metabolic, Template_relevance Pistachio_ringbreaker, Template_relevance Reaxys, Template_relevance Reaxys_biocatalysis model, leveraging a vast database of chemical reactions to predict feasible synthetic routes.
One-Step Synthesis Focus: Specifically designed for one-step synthesis, it provides concise and direct routes for your target compounds, streamlining the synthesis process.
Accurate Predictions: Utilizing the extensive PISTACHIO, BKMS_METABOLIC, PISTACHIO_RINGBREAKER, REAXYS, REAXYS_BIOCATALYSIS database, our tool offers high-accuracy predictions, reflecting the latest in chemical research and data.
Strategy Settings
Precursor scoring | Relevance Heuristic |
---|---|
Min. plausibility | 0.01 |
Model | Template_relevance |
Template Set | Pistachio/Bkms_metabolic/Pistachio_ringbreaker/Reaxys/Reaxys_biocatalysis |
Top-N result to add to graph | 6 |
Feasible Synthetic Routes
Disclaimer and Information on In-Vitro Research Products
Please be aware that all articles and product information presented on BenchChem are intended solely for informational purposes. The products available for purchase on BenchChem are specifically designed for in-vitro studies, which are conducted outside of living organisms. In-vitro studies, derived from the Latin term "in glass," involve experiments performed in controlled laboratory settings using cells or tissues. It is important to note that these products are not categorized as medicines or drugs, and they have not received approval from the FDA for the prevention, treatment, or cure of any medical condition, ailment, or disease. We must emphasize that any form of bodily introduction of these products into humans or animals is strictly prohibited by law. It is essential to adhere to these guidelines to ensure compliance with legal and ethical standards in research and experimentation.