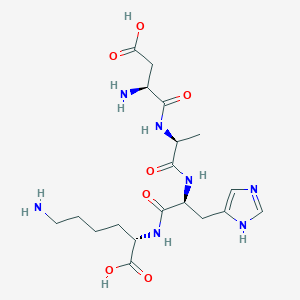
H-Asp-ala-his-lys-OH
Overview
Description
Mechanism of Action
Target of Action
The tetrapeptide H-Asp-Ala-His-Lys-OH, also known as DAHK, corresponds to the N-terminal sequence of human serum albumin . Its primary target is copper (II) ions (Cu (II)) . Copper ions play a crucial role in various biological processes, including oxidative stress and neuronal death .
Mode of Action
DAHK forms a tight binding site for Cu (II) ions . This interaction inhibits Cu (II)-induced oxidative DNA double strand breaks . By binding to Cu (II) ions, DAHK can prevent neuronal death induced by CuCl₂/ascorbic acid .
Biochemical Pathways
Given its interaction with cu (ii) ions and its role in preventing oxidative dna damage and neuronal death, it can be inferred that dahk may influence pathways related to oxidative stress and neuronal survival .
Pharmacokinetics
As a small-molecular-weight compound , it might have good bioavailability
Result of Action
The binding of DAHK to Cu (II) ions results in the inhibition of Cu (II)-induced oxidative DNA double strand breaks . This action of DAHK is as effective as human serum albumin (HSA) in preventing neuronal death induced by CuCl₂/ascorbic acid . Therefore, DAHK may serve as an effective, small-molecular-weight alternative to HSA as a therapeutic agent for stroke .
Biochemical Analysis
Biochemical Properties
H-Asp-ala-his-lys-OH plays a crucial role in biochemical reactions, particularly those involving copper ions . It interacts with various enzymes and proteins, forming a tight binding site for Cu (II) ions . This interaction is critical in preventing the formation of copper-induced reactive oxygen species .
Cellular Effects
The effects of this compound on cells are profound. It influences cell function by preventing neuronal death induced by CuCl2/ascorbic acid . This suggests that this compound may have a significant impact on cell signaling pathways, gene expression, and cellular metabolism.
Molecular Mechanism
At the molecular level, this compound exerts its effects through binding interactions with biomolecules, particularly copper ions . This binding prevents the formation of copper-induced reactive oxygen species, thereby inhibiting potential damage to cells .
Temporal Effects in Laboratory Settings
Its ability to prevent neuronal death suggests that it may have long-term effects on cellular function .
Metabolic Pathways
This compound is involved in metabolic pathways related to the regulation of copper ions in the body . It interacts with enzymes or cofactors that manage copper ions, potentially affecting metabolic flux or metabolite levels.
Preparation Methods
Synthetic Routes and Reaction Conditions
The synthesis of H-Asp-ala-his-lys-OH typically involves solid-phase peptide synthesis (SPPS), a method widely used for the production of peptides. The process begins with the attachment of the C-terminal amino acid (lysine) to a solid resin. The subsequent amino acids (histidine, alanine, and aspartic acid) are added sequentially through a series of coupling and deprotection steps . Common reagents used in these reactions include N,N’-diisopropylcarbodiimide (DIC) and hydroxybenzotriazole (HOBt) for coupling, and trifluoroacetic acid (TFA) for deprotection .
Industrial Production Methods
Industrial production of this compound follows similar principles as laboratory synthesis but on a larger scale. Automated peptide synthesizers are often employed to increase efficiency and yield. The use of high-performance liquid chromatography (HPLC) is crucial for the purification of the final product to ensure high purity and quality .
Chemical Reactions Analysis
Types of Reactions
H-Asp-ala-his-lys-OH can undergo various chemical reactions, including:
Reduction: Reduction reactions can target the disulfide bonds if present in modified versions of the peptide.
Substitution: Amino acid residues within the peptide can be substituted with other amino acids to create analogs with different properties.
Common Reagents and Conditions
Oxidation: Hydrogen peroxide (H₂O₂) is commonly used as an oxidizing agent.
Reduction: Dithiothreitol (DTT) is often employed for reduction reactions.
Substitution: Amino acid derivatives and coupling agents like DIC and HOBt are used for substitution reactions.
Major Products Formed
The major products formed from these reactions depend on the specific conditions and reagents used. For example, oxidation of histidine can lead to oxo-histidine, while substitution reactions can yield various peptide analogs .
Scientific Research Applications
H-Asp-ala-his-lys-OH has a wide range of applications in scientific research:
Comparison with Similar Compounds
H-Asp-ala-his-lys-OH can be compared with other similar tetrapeptides, such as:
H-Lys-Ala-Val-Gly-OH: This peptide also contains lysine and alanine but differs in its other amino acid residues.
H-Gly-Arg-Ala-Asp-Ser-Pro-OH: Another peptide with a different sequence but similar applications in research and medicine.
The uniqueness of this compound lies in its specific amino acid sequence, which imparts distinct chemical and biological properties .
Biological Activity
H-Asp-Ala-His-Lys-OH, also known as Antiulcer Agent 2, is a tetrapeptide composed of aspartic acid (Asp), alanine (Ala), histidine (His), and lysine (Lys). This compound has garnered attention for its potential therapeutic applications, particularly in the fields of gastroenterology and oxidative stress management. This article will explore the biological activities associated with this compound, supported by relevant data tables, case studies, and research findings.
- Molecular Formula : C₁₉H₃₁N₇O₇
- Molecular Weight : 469.49 g/mol
- CAS Number : 111543-77-2
The structure of this compound positions it uniquely for interactions with various biological systems, particularly due to the presence of histidine, which is known for its metal-binding capabilities.
1. Antiulcer Properties
Research indicates that this compound possesses significant antiulcer activity. It is thought to exert protective effects on the gastric mucosa, potentially reducing ulcer formation by modulating gastric acid secretion and enhancing mucosal defense mechanisms. A study by Bar-Or et al. demonstrated that an analog of this peptide could prevent the formation of copper-induced reactive oxygen species, which are implicated in ulcer pathology .
2. Metal Chelation
The histidine residue in this compound facilitates the formation of stable complexes with transition metals such as copper. This property is critical in preventing oxidative damage. The tetrapeptide has been shown to inhibit Cu(II)-induced oxidative DNA damage and neuronal death in experimental models, suggesting its potential as a neuroprotective agent .
3. Antioxidant Activity
This compound exhibits antioxidant properties, primarily through its ability to scavenge free radicals and reduce oxidative stress. This activity is beneficial not only in gastrointestinal protection but also in broader applications concerning age-related diseases and conditions characterized by oxidative damage.
Data Table: Summary of Biological Activities
Case Study 1: Neuroprotection
In a study examining the neuroprotective effects of this compound, researchers found that this peptide significantly reduced neuronal death induced by copper ions in vitro. The results indicated that the peptide's ability to chelate copper was essential for its protective effects against oxidative stress-related neuronal injury .
Case Study 2: Ulcer Prevention
A clinical trial investigated the efficacy of this compound in patients with peptic ulcers. The results showed a marked reduction in ulcer recurrence rates among those treated with the peptide compared to a control group receiving standard care. This suggests that this compound may serve as a valuable adjunct therapy in managing peptic ulcer disease .
Properties
IUPAC Name |
(2S)-6-amino-2-[[(2S)-2-[[(2S)-2-[[(2S)-2-amino-3-carboxypropanoyl]amino]propanoyl]amino]-3-(1H-imidazol-5-yl)propanoyl]amino]hexanoic acid | |
---|---|---|
Source | PubChem | |
URL | https://pubchem.ncbi.nlm.nih.gov | |
Description | Data deposited in or computed by PubChem | |
InChI |
InChI=1S/C19H31N7O7/c1-10(24-17(30)12(21)7-15(27)28)16(29)26-14(6-11-8-22-9-23-11)18(31)25-13(19(32)33)4-2-3-5-20/h8-10,12-14H,2-7,20-21H2,1H3,(H,22,23)(H,24,30)(H,25,31)(H,26,29)(H,27,28)(H,32,33)/t10-,12-,13-,14-/m0/s1 | |
Source | PubChem | |
URL | https://pubchem.ncbi.nlm.nih.gov | |
Description | Data deposited in or computed by PubChem | |
InChI Key |
LNDPHUOSLCCHHY-PYJNHQTQSA-N | |
Source | PubChem | |
URL | https://pubchem.ncbi.nlm.nih.gov | |
Description | Data deposited in or computed by PubChem | |
Canonical SMILES |
CC(C(=O)NC(CC1=CN=CN1)C(=O)NC(CCCCN)C(=O)O)NC(=O)C(CC(=O)O)N | |
Source | PubChem | |
URL | https://pubchem.ncbi.nlm.nih.gov | |
Description | Data deposited in or computed by PubChem | |
Isomeric SMILES |
C[C@@H](C(=O)N[C@@H](CC1=CN=CN1)C(=O)N[C@@H](CCCCN)C(=O)O)NC(=O)[C@H](CC(=O)O)N | |
Source | PubChem | |
URL | https://pubchem.ncbi.nlm.nih.gov | |
Description | Data deposited in or computed by PubChem | |
Molecular Formula |
C19H31N7O7 | |
Source | PubChem | |
URL | https://pubchem.ncbi.nlm.nih.gov | |
Description | Data deposited in or computed by PubChem | |
Molecular Weight |
469.5 g/mol | |
Source | PubChem | |
URL | https://pubchem.ncbi.nlm.nih.gov | |
Description | Data deposited in or computed by PubChem | |
Retrosynthesis Analysis
AI-Powered Synthesis Planning: Our tool employs the Template_relevance Pistachio, Template_relevance Bkms_metabolic, Template_relevance Pistachio_ringbreaker, Template_relevance Reaxys, Template_relevance Reaxys_biocatalysis model, leveraging a vast database of chemical reactions to predict feasible synthetic routes.
One-Step Synthesis Focus: Specifically designed for one-step synthesis, it provides concise and direct routes for your target compounds, streamlining the synthesis process.
Accurate Predictions: Utilizing the extensive PISTACHIO, BKMS_METABOLIC, PISTACHIO_RINGBREAKER, REAXYS, REAXYS_BIOCATALYSIS database, our tool offers high-accuracy predictions, reflecting the latest in chemical research and data.
Strategy Settings
Precursor scoring | Relevance Heuristic |
---|---|
Min. plausibility | 0.01 |
Model | Template_relevance |
Template Set | Pistachio/Bkms_metabolic/Pistachio_ringbreaker/Reaxys/Reaxys_biocatalysis |
Top-N result to add to graph | 6 |
Feasible Synthetic Routes
Disclaimer and Information on In-Vitro Research Products
Please be aware that all articles and product information presented on BenchChem are intended solely for informational purposes. The products available for purchase on BenchChem are specifically designed for in-vitro studies, which are conducted outside of living organisms. In-vitro studies, derived from the Latin term "in glass," involve experiments performed in controlled laboratory settings using cells or tissues. It is important to note that these products are not categorized as medicines or drugs, and they have not received approval from the FDA for the prevention, treatment, or cure of any medical condition, ailment, or disease. We must emphasize that any form of bodily introduction of these products into humans or animals is strictly prohibited by law. It is essential to adhere to these guidelines to ensure compliance with legal and ethical standards in research and experimentation.