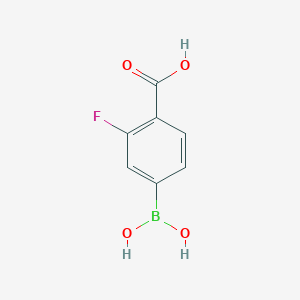
4-Carboxy-3-fluorophenylboronic acid
Overview
Description
Cycloheximide, also known as Naramycin A, is a naturally occurring fungicide produced by the bacterium Streptomyces griseus. It is a well-known inhibitor of protein synthesis in eukaryotic organisms. Cycloheximide is widely used in biomedical research to inhibit protein synthesis in eukaryotic cells studied in vitro. It is inexpensive, works rapidly, and its effects are reversible by simply removing it from the culture medium .
Mechanism of Action
Target of Action
4-Carboxy-3-fluorophenylboronic acid is primarily used as a reagent in the discovery of novel azaindole-based series as potent AXL kinase inhibitors . AXL kinase is a receptor tyrosine kinase that plays essential roles in various cellular processes, including proliferation, survival, and migration .
Mode of Action
The compound interacts with its targets through Suzuki–Miyaura (SM) cross-coupling, a widely-applied transition metal catalysed carbon–carbon bond forming reaction . This process involves the transfer of formally nucleophilic organic groups from boron to palladium .
Biochemical Pathways
It’s known that the compound plays a role in the synthesis of novel azaindole-based series, which can inhibit axl kinase . This suggests that the compound may affect pathways involving AXL kinase.
Pharmacokinetics
The pharmacokinetics of this compound-grafted ε-polylysine (CFPBA-g-PL) have been studied . CFPBA-g-PL has low cytotoxicity, good hemocompatibility, and no tissue reaction . .
Result of Action
The molecular and cellular effects of this compound’s action are largely dependent on its role in the synthesis of novel azaindole-based series . These series can inhibit AXL kinase, potentially affecting cellular processes such as proliferation, survival, and migration .
Action Environment
The action, efficacy, and stability of this compound can be influenced by various environmental factors. For instance, the compound is used under exceptionally mild and functional group tolerant reaction conditions in Suzuki–Miyaura coupling . Additionally, it should be stored in an inert atmosphere at 2-8°C .
Biochemical Analysis
Biochemical Properties
4-Carboxy-3-fluorophenylboronic acid has been used as a reagent in the discovery of novel azaindole-based series as potent AXL kinase inhibitors This suggests that it may interact with enzymes and proteins involved in kinase signaling pathways
Cellular Effects
In the context of cellular effects, this compound has been used in the synthesis of glucose-responsive insulin delivery systems . It has been shown to have low cytotoxicity, good hemocompatibility, and no tissue reaction
Molecular Mechanism
It is known that boronic acids can form reversible covalent complexes with diols, a property that is often exploited in the design of glucose-responsive systems
Temporal Effects in Laboratory Settings
It has been used in the synthesis of glucose-responsive insulin delivery systems, suggesting that it may have long-term effects on cellular function in in vitro or in vivo studies .
Preparation Methods
Cycloheximide is typically produced through fermentation processes involving Streptomyces griseus. The bacterium is cultured in a suitable medium, and cycloheximide is extracted and purified from the culture broth. The synthetic route involves the fermentation of Streptomyces griseus, followed by extraction and purification steps to isolate cycloheximide .
Chemical Reactions Analysis
Cycloheximide undergoes various chemical reactions, including:
Oxidation: Cycloheximide can be oxidized under specific conditions to form different oxidation products.
Reduction: Reduction reactions can convert cycloheximide into its reduced forms.
Substitution: Cycloheximide can undergo substitution reactions where functional groups are replaced by other groups.
Common reagents used in these reactions include oxidizing agents like potassium permanganate, reducing agents like sodium borohydride, and various nucleophiles for substitution reactions. The major products formed from these reactions depend on the specific conditions and reagents used .
Scientific Research Applications
Cycloheximide has a wide range of scientific research applications, including:
Chemistry: Used as a tool to study protein synthesis and its inhibition in eukaryotic cells.
Biology: Employed in experiments to understand the mechanisms of protein synthesis and degradation.
Medicine: Used in research to study the effects of protein synthesis inhibition on various diseases and conditions.
Comparison with Similar Compounds
Cycloheximide is unique in its ability to rapidly and reversibly inhibit protein synthesis in eukaryotic cells. Similar compounds include:
Chloramphenicol: Another protein synthesis inhibitor, but it acts on the 50S ribosomal subunit in prokaryotic cells.
Puromycin: Inhibits protein synthesis by causing premature chain termination during translation.
Anisomycin: Inhibits protein synthesis by binding to the 60S ribosomal subunit, similar to cycloheximide, but with different binding characteristics.
Cycloheximide stands out due to its rapid action and reversibility, making it a valuable tool in research .
Properties
IUPAC Name |
4-borono-2-fluorobenzoic acid | |
---|---|---|
Source | PubChem | |
URL | https://pubchem.ncbi.nlm.nih.gov | |
Description | Data deposited in or computed by PubChem | |
InChI |
InChI=1S/C7H6BFO4/c9-6-3-4(8(12)13)1-2-5(6)7(10)11/h1-3,12-13H,(H,10,11) | |
Source | PubChem | |
URL | https://pubchem.ncbi.nlm.nih.gov | |
Description | Data deposited in or computed by PubChem | |
InChI Key |
CZDWJVSOQOMYGC-UHFFFAOYSA-N | |
Source | PubChem | |
URL | https://pubchem.ncbi.nlm.nih.gov | |
Description | Data deposited in or computed by PubChem | |
Canonical SMILES |
B(C1=CC(=C(C=C1)C(=O)O)F)(O)O | |
Source | PubChem | |
URL | https://pubchem.ncbi.nlm.nih.gov | |
Description | Data deposited in or computed by PubChem | |
Molecular Formula |
C7H6BFO4 | |
Source | PubChem | |
URL | https://pubchem.ncbi.nlm.nih.gov | |
Description | Data deposited in or computed by PubChem | |
DSSTOX Substance ID |
DTXSID90376807 | |
Record name | 4-Carboxy-3-fluorophenylboronic acid | |
Source | EPA DSSTox | |
URL | https://comptox.epa.gov/dashboard/DTXSID90376807 | |
Description | DSSTox provides a high quality public chemistry resource for supporting improved predictive toxicology. | |
Molecular Weight |
183.93 g/mol | |
Source | PubChem | |
URL | https://pubchem.ncbi.nlm.nih.gov | |
Description | Data deposited in or computed by PubChem | |
CAS No. |
120153-08-4 | |
Record name | 4-Borono-2-fluorobenzoic acid | |
Source | ChemIDplus | |
URL | https://pubchem.ncbi.nlm.nih.gov/substance/?source=chemidplus&sourceid=0120153084 | |
Description | ChemIDplus is a free, web search system that provides access to the structure and nomenclature authority files used for the identification of chemical substances cited in National Library of Medicine (NLM) databases, including the TOXNET system. | |
Record name | 4-Carboxy-3-fluorophenylboronic acid | |
Source | EPA DSSTox | |
URL | https://comptox.epa.gov/dashboard/DTXSID90376807 | |
Description | DSSTox provides a high quality public chemistry resource for supporting improved predictive toxicology. | |
Record name | 4-Carboxy-3-fluorobenzeneboronic acid | |
Source | European Chemicals Agency (ECHA) | |
URL | https://echa.europa.eu/information-on-chemicals | |
Description | The European Chemicals Agency (ECHA) is an agency of the European Union which is the driving force among regulatory authorities in implementing the EU's groundbreaking chemicals legislation for the benefit of human health and the environment as well as for innovation and competitiveness. | |
Explanation | Use of the information, documents and data from the ECHA website is subject to the terms and conditions of this Legal Notice, and subject to other binding limitations provided for under applicable law, the information, documents and data made available on the ECHA website may be reproduced, distributed and/or used, totally or in part, for non-commercial purposes provided that ECHA is acknowledged as the source: "Source: European Chemicals Agency, http://echa.europa.eu/". Such acknowledgement must be included in each copy of the material. ECHA permits and encourages organisations and individuals to create links to the ECHA website under the following cumulative conditions: Links can only be made to webpages that provide a link to the Legal Notice page. | |
Record name | 4-Borono-2-fluorobenzoic acid | |
Source | FDA Global Substance Registration System (GSRS) | |
URL | https://gsrs.ncats.nih.gov/ginas/app/beta/substances/MT2PH75ZYW | |
Description | The FDA Global Substance Registration System (GSRS) enables the efficient and accurate exchange of information on what substances are in regulated products. Instead of relying on names, which vary across regulatory domains, countries, and regions, the GSRS knowledge base makes it possible for substances to be defined by standardized, scientific descriptions. | |
Explanation | Unless otherwise noted, the contents of the FDA website (www.fda.gov), both text and graphics, are not copyrighted. They are in the public domain and may be republished, reprinted and otherwise used freely by anyone without the need to obtain permission from FDA. Credit to the U.S. Food and Drug Administration as the source is appreciated but not required. | |
Retrosynthesis Analysis
AI-Powered Synthesis Planning: Our tool employs the Template_relevance Pistachio, Template_relevance Bkms_metabolic, Template_relevance Pistachio_ringbreaker, Template_relevance Reaxys, Template_relevance Reaxys_biocatalysis model, leveraging a vast database of chemical reactions to predict feasible synthetic routes.
One-Step Synthesis Focus: Specifically designed for one-step synthesis, it provides concise and direct routes for your target compounds, streamlining the synthesis process.
Accurate Predictions: Utilizing the extensive PISTACHIO, BKMS_METABOLIC, PISTACHIO_RINGBREAKER, REAXYS, REAXYS_BIOCATALYSIS database, our tool offers high-accuracy predictions, reflecting the latest in chemical research and data.
Strategy Settings
Precursor scoring | Relevance Heuristic |
---|---|
Min. plausibility | 0.01 |
Model | Template_relevance |
Template Set | Pistachio/Bkms_metabolic/Pistachio_ringbreaker/Reaxys/Reaxys_biocatalysis |
Top-N result to add to graph | 6 |
Feasible Synthetic Routes
Q1: How does 4-Carboxy-3-fluorophenylboronic acid interact with glucose and what are the downstream effects?
A1: this compound (FPBA) reversibly binds to glucose through its boronic acid moiety, forming a cyclic ester. [, , , , , , , ] This binding event triggers a change in the physicochemical properties of FPBA-containing materials. For example, it can lead to a shift in the material's charge from neutral to negative, increase its hydrophilicity, or induce disassembly of self-assembled structures. [, , , ] These changes can be harnessed for glucose-responsive drug release.
Q2: What is the impact of the fluorine atom in this compound compared to the non-fluorinated analog?
A2: The fluorine atom in FPBA increases its acidity compared to 4-carboxyphenylboronic acid (PBA). [, ] This results in a lower pKa value for FPBA, enabling it to bind glucose more effectively at physiological pH. [] Consequently, FPBA-based systems often exhibit enhanced glucose sensitivity and responsiveness compared to PBA-based counterparts. [, ]
Q3: What are some examples of how this compound has been incorporated into glucose-responsive drug delivery systems?
A3: FPBA has been incorporated into various drug delivery systems, including:
- Polyplex micelles: FPBA-modified polyplex micelles have been developed for both gene [, ] and peptide delivery. [] These micelles exhibit glucose-responsive changes in their core crosslinking, leading to controlled release of the therapeutic payload.
- Nanoparticles: FPBA-modified nanoparticles have been designed for insulin delivery. [, , ] The nanoparticles release insulin in response to elevated glucose levels, mimicking the function of pancreatic beta cells.
- Microneedles: Biodegradable microneedles encapsulating FPBA-modified particles have been explored for transdermal insulin delivery. [] These microneedles offer a minimally invasive approach for glucose-responsive insulin administration.
Q4: What are the potential advantages of using this compound in glucose-responsive drug delivery systems?
A4: FPBA offers several advantages for glucose-responsive drug delivery, including:
- Biocompatibility: FPBA-modified materials have demonstrated good biocompatibility in several studies. [, , ]
- Tunable Responsiveness: The glucose sensitivity and response kinetics of FPBA-based systems can be fine-tuned by adjusting factors like the degree of FPBA modification and the overall material composition. [, , ]
- Versatility: FPBA can be conjugated to various polymers, lipids, and nanoparticles, enabling the development of diverse glucose-responsive drug delivery platforms. [, , , , , ]
Q5: What are the limitations or challenges associated with using this compound in drug delivery systems?
A5: Some challenges associated with using FPBA include:
- Long-term stability: The long-term stability of FPBA-modified materials and their potential for degradation in vivo requires further investigation. []
- Immunogenicity: The potential immunogenicity of FPBA-containing systems, particularly with repeated administration, needs to be carefully assessed. []
Q6: What are the future directions for research on this compound in drug delivery?
A6: Future research could focus on:
Disclaimer and Information on In-Vitro Research Products
Please be aware that all articles and product information presented on BenchChem are intended solely for informational purposes. The products available for purchase on BenchChem are specifically designed for in-vitro studies, which are conducted outside of living organisms. In-vitro studies, derived from the Latin term "in glass," involve experiments performed in controlled laboratory settings using cells or tissues. It is important to note that these products are not categorized as medicines or drugs, and they have not received approval from the FDA for the prevention, treatment, or cure of any medical condition, ailment, or disease. We must emphasize that any form of bodily introduction of these products into humans or animals is strictly prohibited by law. It is essential to adhere to these guidelines to ensure compliance with legal and ethical standards in research and experimentation.