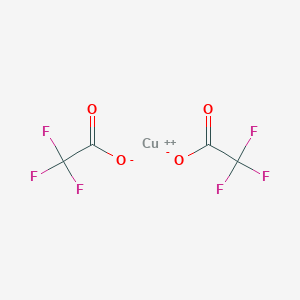
Copper (II) Trifluoroacetate
- Click on QUICK INQUIRY to receive a quote from our team of experts.
- With the quality product at a COMPETITIVE price, you can focus more on your research.
Overview
Description
Copper (II) Trifluoroacetate is a chemical compound with the formula Cu(CF₃COO)₂. It is the trifluoroacetate of divalent copper and exists in various forms, including anhydrous, hydrate, and adducts with other solvents. The compound is known for its blue crystalline appearance and is soluble in water .
Preparation Methods
Copper (II) Trifluoroacetate can be synthesized by reacting trifluoroacetic acid with copper oxide, copper hydroxide, or basic copper carbonate. The reaction typically involves the following steps:
Reaction with Copper Oxide: Trifluoroacetic acid reacts with copper oxide to form this compound.
Reaction with Copper Hydroxide: Trifluoroacetic acid reacts with copper hydroxide to produce this compound.
Reaction with Basic Copper Carbonate: Trifluoroacetic acid reacts with basic copper carbonate to yield this compound.
Industrial production methods often involve the use of acetone to replace water molecules in the hydrate form of this compound. Under reduced pressure conditions, acetone can be removed to obtain the anhydrous material .
Chemical Reactions Analysis
Copper (II) Trifluoroacetate undergoes various types of chemical reactions, including:
Oxidation: this compound can be oxidized to form copper (III) compounds.
Reduction: The compound can be reduced to form copper (I) compounds.
Substitution: this compound forms adducts with a variety of Lewis bases, such as ammonia, water, dioxane, and quinoline.
Common reagents and conditions used in these reactions include trifluoroacetic acid, copper oxide, copper hydroxide, and basic copper carbonate. Major products formed from these reactions include copper (I) nitride and other copper-based compounds .
Scientific Research Applications
Copper (II) Trifluoroacetate has a wide range of scientific research applications, including:
Biology: The compound is used in various biochemical assays and studies involving copper-based enzymes.
Medicine: this compound is explored for its potential therapeutic applications, including its use in drug delivery systems.
Mechanism of Action
The mechanism of action of Copper (II) Trifluoroacetate involves its ability to form adducts with Lewis bases. The Lewis bases bind to the axial positions of the copper center, facilitating various chemical reactions. The compound’s effects are exerted through its interactions with molecular targets, including enzymes and other proteins involved in biochemical pathways .
Comparison with Similar Compounds
Copper (II) Trifluoroacetate can be compared with other similar compounds, such as:
Copper (II) Acetate: Similar to this compound, Copper (II) Acetate is used in organic synthesis and as a precursor for other copper-based compounds.
Copper (II) Trifluoromethanesulfonate: This compound is used in similar applications, including as a reagent in organic synthesis.
Copper (II) Acetylacetonate: Used in various industrial applications, including as a catalyst and in the production of other copper-based materials.
This compound is unique due to its trifluoroacetate ligand, which imparts distinct chemical properties and reactivity compared to other copper-based compounds.
Properties
CAS No. |
123333-88-0 |
---|---|
Molecular Formula |
C2H3CuF3O3 |
Molecular Weight |
195.58 g/mol |
IUPAC Name |
copper;2,2,2-trifluoroacetic acid;hydrate |
InChI |
InChI=1S/C2HF3O2.Cu.H2O/c3-2(4,5)1(6)7;;/h(H,6,7);;1H2 |
InChI Key |
AKQGQKFVDGRGJP-UHFFFAOYSA-N |
SMILES |
C(=O)(C(F)(F)F)[O-].C(=O)(C(F)(F)F)[O-].[Cu+2] |
Canonical SMILES |
C(=O)(C(F)(F)F)O.O.[Cu] |
Pictograms |
Irritant |
Origin of Product |
United States |
Retrosynthesis Analysis
AI-Powered Synthesis Planning: Our tool employs the Template_relevance Pistachio, Template_relevance Bkms_metabolic, Template_relevance Pistachio_ringbreaker, Template_relevance Reaxys, Template_relevance Reaxys_biocatalysis model, leveraging a vast database of chemical reactions to predict feasible synthetic routes.
One-Step Synthesis Focus: Specifically designed for one-step synthesis, it provides concise and direct routes for your target compounds, streamlining the synthesis process.
Accurate Predictions: Utilizing the extensive PISTACHIO, BKMS_METABOLIC, PISTACHIO_RINGBREAKER, REAXYS, REAXYS_BIOCATALYSIS database, our tool offers high-accuracy predictions, reflecting the latest in chemical research and data.
Strategy Settings
Precursor scoring | Relevance Heuristic |
---|---|
Min. plausibility | 0.01 |
Model | Template_relevance |
Template Set | Pistachio/Bkms_metabolic/Pistachio_ringbreaker/Reaxys/Reaxys_biocatalysis |
Top-N result to add to graph | 6 |
Feasible Synthetic Routes
A: While the provided research doesn't define a specific biological target for Copper(II) Trifluoroacetate, its interaction often stems from Copper(II)'s ability to coordinate with electron-rich sites in organic molecules. For example, in [], Copper(II) Trifluoroacetate acts as a catalyst by coordinating with carbonyl groups in cycloalkanones, facilitating aldol condensation reactions. The downstream effects are dependent on the specific reaction and substrates involved.
ANone:
- Molecular Formula: Anhydrous form: C4CuF6O4 []; Hydrated form: C4CuH2F6O4·xH2O []
- Molecular Weight: Anhydrous: 289.58 g/mol []; Hydrated: Variable depending on the degree of hydration []
- Spectroscopic Data: Characterization often includes:
- UV-Vis Spectroscopy: Reveals d-d transitions characteristic of Copper(II) complexes, providing information about coordination geometry. [, , ]
- Infrared Spectroscopy (IR): Identifies characteristic stretching frequencies for carboxylate groups and other ligands present. [, ]
- Electron Paramagnetic Resonance (EPR): Provides information about the electronic structure and coordination environment of the Copper(II) ion. [, ]
A: Copper(II) Trifluoroacetate exhibits air and moisture sensitivity, requiring storage under an inert atmosphere. [] It demonstrates volatility, enabling its use in chemical vapor deposition for thin copper film production. [] It acts as a precursor for Copper Selenide nanoparticles in reactions with specific organoselenium compounds. []
ANone: Copper(II) Trifluoroacetate serves as a Lewis acid catalyst in various organic reactions.
- Mechanism: It typically functions by activating electrophilic substrates through coordination, facilitating nucleophilic attack. [, ]
- Selectivity: Observed selectivity depends on the specific reaction. For instance, in crossed-aldol condensation, it shows selectivity towards the formation of α,α′-bis(substituted benzylidene) cycloalkanones. []
- Uses:
A: Yes, "broken symmetry" Density Functional Theory (DFT) calculations have been employed to estimate the exchange integrals in tetranuclear Copper(II) trifluoroacetate complexes. [] These calculations help elucidate magnetic properties and provide insights into the electronic structure of these complexes.
A: Copper(II) Trifluoroacetate is sensitive to moisture; therefore, storage under an inert atmosphere is crucial for maintaining its stability. [] While specific formulation strategies are not discussed, researchers often employ anhydrous solvents and inert conditions when handling this compound.
ANone: While specific historical milestones aren't detailed in the provided research, studies on Copper(II) Trifluoroacetate have contributed to:
- Understanding the coordination chemistry of Copper(II) with perfluorinated carboxylates [, , ].
- Exploring its use as a precursor for material science applications, like the synthesis of copper selenide nanoparticles and thin films [, ].
- Investigating its catalytic activity in organic synthesis, expanding the repertoire of copper-catalyzed reactions [, , , ].
ANone: The research on Copper(II) Trifluoroacetate showcases interdisciplinary connections:
- Coordination Chemistry and Materials Science: Synthesis of copper selenide nanoparticles [] and copper nitride powders/films [] relies on understanding the reactivity and decomposition pathways of Copper(II) Trifluoroacetate.
- Inorganic Chemistry and Catalysis: Developing Copper(II) Trifluoroacetate-catalyzed organic reactions like [3+2] annulations [] and crossed-aldol condensations [] necessitates understanding both the coordination chemistry and catalytic mechanisms.
- Synthetic Chemistry and Spectroscopy: Characterizing the structure and properties of Copper(II) Trifluoroacetate complexes often involves a synergistic combination of synthesis, spectroscopic techniques (UV-Vis, IR, EPR), and computational modeling [, , , ].
Disclaimer and Information on In-Vitro Research Products
Please be aware that all articles and product information presented on BenchChem are intended solely for informational purposes. The products available for purchase on BenchChem are specifically designed for in-vitro studies, which are conducted outside of living organisms. In-vitro studies, derived from the Latin term "in glass," involve experiments performed in controlled laboratory settings using cells or tissues. It is important to note that these products are not categorized as medicines or drugs, and they have not received approval from the FDA for the prevention, treatment, or cure of any medical condition, ailment, or disease. We must emphasize that any form of bodily introduction of these products into humans or animals is strictly prohibited by law. It is essential to adhere to these guidelines to ensure compliance with legal and ethical standards in research and experimentation.