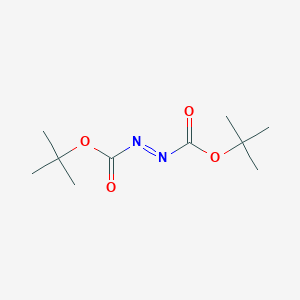
Di-tert-butyl azodicarboxylate
Overview
Description
Di-tert-butyl azodicarboxylate (DTBAD, CAS 870-50-8), also referred to as DBAD , is an azo compound with the molecular formula C₁₀H₁₈N₂O₄ and a molecular weight of 230.26 g/mol . It is characterized by two tert-butyl ester groups attached to an azo (-N=N-) backbone, which confers significant steric bulk and thermal stability. DTBAD is a yellow crystalline solid with a melting point of 90–92°C and low water solubility .
DTBAD is widely employed in organic synthesis, particularly in:
- Asymmetric α-amination: It serves as an electrophilic nitrogen source in enantioselective reactions, achieving high enantioselectivity in organocatalyzed systems .
- Oxidative dehydrogenation: Copper-catalyzed aerobic oxidation of DTBAD derivatives enables mild dehydrogenation of N-heterocycles like tetrahydroquinolines .
- Radical reactions: Its role as a radical acceptor stabilizes intermediates in multicomponent carboamination reactions .
- Thermal hazards: Studies using C80 microcalorimetry and STA-FTIR-MS reveal its exothermic decomposition mechanism, critical for industrial safety protocols .
Preparation Methods
Synthetic Routes and Reaction Conditions
Di-tert-butyl azodicarboxylate can be synthesized through the reaction of di-tert-butyl dicarbonate with hydrazine. The reaction typically involves the use of a solvent such as tetrahydrofuran and is carried out under controlled temperature conditions to ensure the desired product is obtained.
Industrial Production Methods
In industrial settings, the production of this compound often involves large-scale batch processes. The reaction conditions are optimized to maximize yield and purity, and the product is typically purified through recrystallization or distillation.
Chemical Reactions Analysis
Mitsunobu Reactions
DBAD serves as a critical azo reagent in Mitsunobu reactions, facilitating the conversion of alcohols to esters, ethers, or amines with inversion of stereochemistry. Its mechanism involves:
-
Formation of a betaine intermediate with triphenylphosphine (TPP)
-
Activation of the alcohol via proton transfer
Example Reaction:
textMBH alcohol + DBAD + TPP → SN2' product (90% yield)[8]
Comparison of Azodicarboxylates in Mitsunobu Reactions
Reagent | Melting Point (°C) | Nitrogen Content (%) | Thermal Stability (°C) |
---|---|---|---|
DBAD | 89–92 | 12.17 | Decomposes at 113–129 |
DEAD | <0 (liquid) | 16.09 | Decomposes at 96–167 |
DIAD | 88–90 | 13.85 | Decomposes at 186–212 |
Electrophilic Amination
DBAD enables α-amination of carbonyl compounds via organocatalytic pathways:
β-Keto Esters
textβ-keto ester + DBAD → α-aminated product
Nitroacetates
-
Catalyst : β-ICD (Hatakeyama catalyst, 10 mol%)
-
Scope : α-Monosubstituted nitroacetates
Dehydrogenation Reactions
DBAD participates in copper-catalyzed aerobic dehydrogenation:
Tetrahydroquinoline → Quinoline
-
Catalytic System : CuI (10 mol%) + DBAD (1.2 equiv)
-
Conditions : O₂ atmosphere, 60°C, 12 h
Mechanistic Pathway:
-
DBAD oxidizes tetrahydroquinoline to quinoline
-
Reduced DBAD (di-tert-butyl hydrazodicarboxylate) is reoxidized by O₂
Enolate Functionalization
-
Application : Synthesis of peptidomimetics
-
Reaction : Enolate amination of cyclic ketones
Asymmetric Friedel-Crafts
Scientific Research Applications
Catalytic Applications
2.1 Cu-Catalyzed Aerobic Oxidation
DBAD has been employed in a Cu-catalyzed aerobic oxidation process for the dehydrogenation of tetrahydroquinolines. This method allows for the efficient synthesis of quinolines under mild conditions, showcasing DBAD's role as a co-catalyst that facilitates the regeneration of active species through aerobic oxidative processes .
2.2 Asymmetric Amination Reactions
DBAD serves as a practical electrophilic nitrogen source in asymmetric amination reactions. A notable study demonstrated its use in the direct catalytic enantioselective amination of unactivated aryl and vinyl ketones. The reaction was catalyzed by a Zn–ProPhenol system, yielding enantioenriched hydrazine products that can be converted into valuable amino compounds .
Synthetic Methodologies
3.1 Mitsunobu Reaction
DBAD is extensively used in Mitsunobu reactions, where it facilitates the conversion of alcohols into various functional groups. This reaction is significant for synthesizing complex molecules, including chiral compounds .
3.2 Synthesis of Peptidomimetics
The compound has been applied in the synthesis of peptidomimetic compounds via stereoselective N-acyliminium-mediated cyclization and enolate amination. This application highlights DBAD's relevance in pharmaceutical chemistry, particularly in developing peptide-based drugs .
Case Study: Asymmetric Michael Addition Reactions
In a study focusing on asymmetric Michael addition reactions, DBAD was used to enhance selectivity and yield. The results indicated that employing DBAD significantly improved the enantioselectivity of the reaction compared to traditional methods .
Reaction Type | Yield (%) | Enantiomeric Excess (%) |
---|---|---|
Asymmetric Michael Addition | 79 | 79 |
Direct Amination Reaction | 66 | 88 |
Case Study: Preparation of Hexapeptide Fragments
DBAD was utilized for preparing hexapeptide key fragments through hydrazination and cyclization reactions. This method demonstrates its utility in peptide synthesis, allowing for the construction of complex peptide structures with high stereochemical fidelity .
Mechanism of Action
The mechanism of action of di-tert-butyl azodicarboxylate involves its role as an electrophilic reagent. In the Mitsunobu reaction, it facilitates the formation of a good leaving group from an alcohol, which is then displaced by a nucleophile. The reaction proceeds through the formation of a betaine intermediate and involves the reduction of the azo group to a hydrazine derivative.
Comparison with Similar Compounds
Comparison with Similar Azodicarboxylates
Azodicarboxylates share the azo functional group but differ in ester substituents, influencing steric bulk, solubility, and reactivity. Key compounds include diethyl azodicarboxylate (DEAD) , diisopropyl azodicarboxylate (DIAD) , and di-2-methoxyethyl azodicarboxylate (DMEAD) .
Structural and Functional Differences
Reactivity and Selectivity
- Steric Effects : DTBAD’s tert-butyl groups impose high steric hindrance, favoring enantioselectivity in α-amination. For example, in ChCl/glycerol deep eutectic solvents, DTBAD achieved superior enantiomeric excess (e.g., 92% ee) compared to less bulky analogs .
- Safety : DEAD is commercially available in solution due to its explosive nature , whereas DTBAD’s solid-state form reduces handling risks despite its exothermic decomposition above 120°C .
- Reaction Scope: DTBAD is ineffective in classical Mitsunobu reactions due to steric limitations, unlike DEAD/DIAD . However, it excels in copper-catalyzed aerobic oxidations and radical-mediated processes .
Research Findings and Industrial Relevance
- Asymmetric Catalysis : DTBAD’s steric bulk enables high enantioselectivity (up to 96% ee) in Hatakeyama’s β-ICD-catalyzed amination of nitroacetates .
- Radical Stabilization: DFT studies show DTBAD stabilizes radical intermediates in bicyclo[1.1.1]pentylamine synthesis, enabling novel bioisosteres for medicinal chemistry .
- Thermal Hazard Mitigation : DTBAD’s decomposition kinetics (activation energy ~110 kJ/mol) inform safe storage and transport protocols .
Biological Activity
Di-tert-butyl azodicarboxylate (DTBAD), with the chemical formula and CAS number 870-50-8, is a versatile compound widely utilized in organic synthesis. Its biological activity is of significant interest due to its applications in various chemical reactions, particularly in the fields of medicinal chemistry and biochemistry. This article delves into the biological activity of DTBAD, examining its mechanisms, applications, and relevant case studies.
DTBAD is characterized by its yellow crystalline appearance and a molecular weight of 230.26 g/mol. It is an acid-labile reagent commonly employed in Mitsunobu reactions and other electrophilic amination processes. The compound's structure includes two tert-butyl groups attached to an azodicarboxylate moiety, which contributes to its reactivity and stability under various conditions.
Property | Value |
---|---|
Molecular Formula | C10H18N2O4 |
Molecular Weight | 230.26 g/mol |
Appearance | Yellow crystals |
CAS Number | 870-50-8 |
Synonyms | di-tert-butyl diazene-1,2-dicarboxylate |
DTBAD's biological activity is primarily attributed to its role as an electrophilic reagent in various synthetic pathways. It facilitates the generation of reactive intermediates that can engage in further chemical transformations, including:
- Amination Reactions : DTBAD is utilized in electrophilic amination processes, where it reacts with beta-keto esters to form amines under the catalysis of chiral guanidines .
- Hydrazination : The compound serves as a precursor in hydrazination reactions, which are essential for synthesizing hydrazones and related compounds .
Case Studies
-
Cu-Catalyzed Aerobic Oxidation :
A study demonstrated the use of DTBAD in a Cu-catalyzed aerobic oxidation system for synthesizing 1,2,3,4-tetrahydroquinolines. This method showcased DTBAD's ability to regenerate itself from di-tert-butyl hydrazodicarboxylate using molecular oxygen as a terminal oxidant, highlighting its efficiency in organic synthesis . -
Mitsunobu Reaction :
The Mitsunobu reaction involving DTBAD was employed to synthesize cyclic ethers effectively. This reaction underscores the compound's utility in forming complex molecular architectures important for drug development . -
Histone Acetyltransferase Inhibition :
Recent research has explored the potential of DTBAD derivatives as selective inhibitors for histone acetyltransferases (HATs). These enzymes play crucial roles in gene regulation and cancer progression. The development of small molecule inhibitors based on DTBAD could provide new therapeutic avenues for treating cancers driven by aberrant HAT activity .
Table 2: Summary of Biological Applications
Application | Description |
---|---|
Electrophilic Amination | Reacts with beta-keto esters to form amines |
Hydrazination | Serves as a precursor for hydrazone synthesis |
Cu-Catalyzed Oxidation | Facilitates aerobic oxidation of tetrahydroquinolines |
HAT Inhibition | Potential therapeutic target for cancer treatment |
Q & A
Basic Research Questions
Q. What are the optimal methods for synthesizing and purifying DBAD with high yield and purity?
DBAD is typically synthesized via oxidation of di-tert-butyl hydrazodicarboxylate. A Cu-catalyzed aerobic oxidation method achieves moderate yields (38%) under mild conditions, using oxygen as the terminal oxidant . For purification, crystallization from ether at low temperatures (-78°C) is effective, though recrystallization from acetone or ether may require optimization due to challenges in solid product formation . Key parameters include reaction time (e.g., 18 hours for completion) and inert atmosphere handling to prevent premature decomposition .
Q. What safety protocols are critical when handling DBAD in laboratory settings?
- Storage : Store at 2–8°C in a cool, dry place away from heat and ignition sources .
- Personal Protective Equipment (PPE) : Use chemically resistant gloves (EN 374 compliant), flame-retardant lab coats, and eye protection. Gloves must be removed without touching the outer surface to avoid contamination .
- Incompatibilities : Avoid contact with oxidizing agents, reducing agents, acids, and bases. Hazardous decomposition products include carbon oxides under fire conditions .
Q. What is the role of DBAD in Mitsunobu reactions, and how can its efficiency be maximized?
DBAD acts as an oxidizing agent in Mitsunobu reactions, facilitating the transfer of a proton from the substrate to triphenylphosphine. To optimize efficiency:
- Use stoichiometric amounts (e.g., 1.2 equivalents relative to the substrate).
- Ensure anhydrous conditions to prevent hydrolysis.
- Monitor reaction progress via TLC or HPLC, as over-stirring may lead to side reactions. Post-reaction, filter through a glass frit and wash with ethyl acetate to remove phosphine oxide byproducts .
Advanced Research Questions
Q. What are the thermal decomposition mechanisms of DBAD, and how can associated hazards be mitigated?
DBAD undergoes exothermic decomposition above 120°C, releasing gases such as CO, CO₂, and tert-butoxy radicals. Studies using C80 microcalorimetry and STA-FTIR-MS reveal a two-stage decomposition pathway:
Initial endothermic phase (90–120°C): Molecular rearrangement.
Exothermic phase (>120°C): Radical chain reactions .
Mitigation Strategies :
- Conduct reactions below 100°C.
- Use pressure-relief devices in closed systems.
- Avoid sudden temperature spikes during scale-up .
Q. How does DBAD participate in photocatalytic C–N bond formation, and what mechanistic insights exist?
In FeCl₃-photocatalyzed reactions, DBAD acts as an alkyl radical acceptor. Under 365 nm LED irradiation, FeCl₃·6H₂O generates α-amino radicals from light alkanes, which couple with DBAD-derived radical anions to form C–N bonds. Key steps:
- Photoexcitation of FeCl₃ generates reactive intermediates.
- DBAD is reduced to a radical anion, enabling radical-radical coupling.
- Turnover numbers (TONs) up to 8000 have been achieved, highlighting scalability .
Q. How should researchers address contradictions in experimental data, such as inconsistent yields or side products?
Case Example : A study reported 38% yield for DBAD synthesis via crystallization , while catalytic methods achieved higher efficiency . Resolution Strategies :
Properties
IUPAC Name |
tert-butyl (NE)-N-[(2-methylpropan-2-yl)oxycarbonylimino]carbamate | |
---|---|---|
Source | PubChem | |
URL | https://pubchem.ncbi.nlm.nih.gov | |
Description | Data deposited in or computed by PubChem | |
InChI |
InChI=1S/C10H18N2O4/c1-9(2,3)15-7(13)11-12-8(14)16-10(4,5)6/h1-6H3/b12-11+ | |
Source | PubChem | |
URL | https://pubchem.ncbi.nlm.nih.gov | |
Description | Data deposited in or computed by PubChem | |
InChI Key |
QKSQWQOAUQFORH-VAWYXSNFSA-N | |
Source | PubChem | |
URL | https://pubchem.ncbi.nlm.nih.gov | |
Description | Data deposited in or computed by PubChem | |
Canonical SMILES |
CC(C)(C)OC(=O)N=NC(=O)OC(C)(C)C | |
Source | PubChem | |
URL | https://pubchem.ncbi.nlm.nih.gov | |
Description | Data deposited in or computed by PubChem | |
Isomeric SMILES |
CC(C)(C)OC(=O)/N=N/C(=O)OC(C)(C)C | |
Source | PubChem | |
URL | https://pubchem.ncbi.nlm.nih.gov | |
Description | Data deposited in or computed by PubChem | |
Molecular Formula |
C10H18N2O4 | |
Source | PubChem | |
URL | https://pubchem.ncbi.nlm.nih.gov | |
Description | Data deposited in or computed by PubChem | |
Molecular Weight |
230.26 g/mol | |
Source | PubChem | |
URL | https://pubchem.ncbi.nlm.nih.gov | |
Description | Data deposited in or computed by PubChem | |
CAS No. |
870-50-8 | |
Record name | Di-tert-butyl azodicarboxylate | |
Source | ChemIDplus | |
URL | https://pubchem.ncbi.nlm.nih.gov/substance/?source=chemidplus&sourceid=0000870508 | |
Description | ChemIDplus is a free, web search system that provides access to the structure and nomenclature authority files used for the identification of chemical substances cited in National Library of Medicine (NLM) databases, including the TOXNET system. | |
Record name | 870-50-8 | |
Source | DTP/NCI | |
URL | https://dtp.cancer.gov/dtpstandard/servlet/dwindex?searchtype=NSC&outputformat=html&searchlist=109889 | |
Description | The NCI Development Therapeutics Program (DTP) provides services and resources to the academic and private-sector research communities worldwide to facilitate the discovery and development of new cancer therapeutic agents. | |
Explanation | Unless otherwise indicated, all text within NCI products is free of copyright and may be reused without our permission. Credit the National Cancer Institute as the source. | |
Record name | Di-tert-butyl azodicarboxylate | |
Source | European Chemicals Agency (ECHA) | |
URL | https://echa.europa.eu/substance-information/-/substanceinfo/100.011.634 | |
Description | The European Chemicals Agency (ECHA) is an agency of the European Union which is the driving force among regulatory authorities in implementing the EU's groundbreaking chemicals legislation for the benefit of human health and the environment as well as for innovation and competitiveness. | |
Explanation | Use of the information, documents and data from the ECHA website is subject to the terms and conditions of this Legal Notice, and subject to other binding limitations provided for under applicable law, the information, documents and data made available on the ECHA website may be reproduced, distributed and/or used, totally or in part, for non-commercial purposes provided that ECHA is acknowledged as the source: "Source: European Chemicals Agency, http://echa.europa.eu/". Such acknowledgement must be included in each copy of the material. ECHA permits and encourages organisations and individuals to create links to the ECHA website under the following cumulative conditions: Links can only be made to webpages that provide a link to the Legal Notice page. | |
Retrosynthesis Analysis
AI-Powered Synthesis Planning: Our tool employs the Template_relevance Pistachio, Template_relevance Bkms_metabolic, Template_relevance Pistachio_ringbreaker, Template_relevance Reaxys, Template_relevance Reaxys_biocatalysis model, leveraging a vast database of chemical reactions to predict feasible synthetic routes.
One-Step Synthesis Focus: Specifically designed for one-step synthesis, it provides concise and direct routes for your target compounds, streamlining the synthesis process.
Accurate Predictions: Utilizing the extensive PISTACHIO, BKMS_METABOLIC, PISTACHIO_RINGBREAKER, REAXYS, REAXYS_BIOCATALYSIS database, our tool offers high-accuracy predictions, reflecting the latest in chemical research and data.
Strategy Settings
Precursor scoring | Relevance Heuristic |
---|---|
Min. plausibility | 0.01 |
Model | Template_relevance |
Template Set | Pistachio/Bkms_metabolic/Pistachio_ringbreaker/Reaxys/Reaxys_biocatalysis |
Top-N result to add to graph | 6 |
Feasible Synthetic Routes
Disclaimer and Information on In-Vitro Research Products
Please be aware that all articles and product information presented on BenchChem are intended solely for informational purposes. The products available for purchase on BenchChem are specifically designed for in-vitro studies, which are conducted outside of living organisms. In-vitro studies, derived from the Latin term "in glass," involve experiments performed in controlled laboratory settings using cells or tissues. It is important to note that these products are not categorized as medicines or drugs, and they have not received approval from the FDA for the prevention, treatment, or cure of any medical condition, ailment, or disease. We must emphasize that any form of bodily introduction of these products into humans or animals is strictly prohibited by law. It is essential to adhere to these guidelines to ensure compliance with legal and ethical standards in research and experimentation.