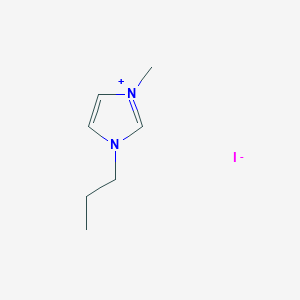
1-Methyl-3-propylimidazolium iodide
Overview
Description
1-Methyl-3-propylimidazolium iodide is a type of ionic liquid compound, characterized by its unique properties such as high thermal stability and electrical conductivity. It is a colorless solid that is soluble in water and various organic solvents
Mechanism of Action
Target of Action
1-Methyl-3-propylimidazolium iodide (MPII) is an ionic liquid compound . Its primary targets are the chemical reactions and processes where it acts as a solvent, electrolyte, and catalyst .
Mode of Action
MPII interacts with its targets by facilitating chemical reactions and processes. It provides a medium for reactions to occur, enhances the conductivity of the system, and accelerates the reaction rate .
Biochemical Pathways
MPII affects various biochemical pathways, including catalytic reactions, ionic liquid electrolytes, metal electrodeposition, electrochemical synthesis, and organic synthesis . The downstream effects of these pathways include enhanced reaction rates, improved conductivity, and efficient synthesis of desired products .
Result of Action
The molecular and cellular effects of MPII’s action are primarily observed in its role as a solvent, electrolyte, and catalyst. It contributes to the successful completion of chemical reactions and processes, leading to the efficient production of desired outcomes .
Biochemical Analysis
Biochemical Properties
It is known that it can be used in catalytic reactions, ionic liquid electrolytes, metal electrodeposition, electrochemical synthesis, and organic synthesis . It can serve as a solvent, electrolyte, and alternative to catalysts, and is widely used in chemical reactions and material synthesis .
Cellular Effects
It has been used in dye-sensitized nanocrystalline titanium dioxide (TiO2) solar cells
Molecular Mechanism
It is known to interact with other molecules in the context of dye-sensitized solar cells
Temporal Effects in Laboratory Settings
It is known to have good thermal stability , suggesting that it may have stable effects over time
Preparation Methods
1-Methyl-3-propylimidazolium iodide can be synthesized through the reaction of 1-methylimidazole with propyl iodide . The reaction typically occurs under solvent-free conditions in a stainless steel autoclave lined with polytetrafluoroethylene, ensuring high purity and nearly complete conversion of 1-methylimidazole . This method is not only efficient but also environmentally friendly.
Chemical Reactions Analysis
1-Methyl-3-propylimidazolium iodide undergoes various chemical reactions, including:
Substitution Reactions: It can participate in nucleophilic substitution reactions where the iodide ion is replaced by other nucleophiles.
Oxidation and Reduction: The compound can be involved in redox reactions, particularly in electrochemical applications.
Complex Formation: It can form complexes with various metal ions, which is useful in catalysis and material science.
Common reagents used in these reactions include halides, oxidizing agents, and reducing agents. The major products formed depend on the specific reaction conditions and reagents used.
Scientific Research Applications
Corrosion Inhibition
One of the prominent applications of MPII is in the field of corrosion inhibition. Research has demonstrated its effectiveness in protecting mild steel from corrosion in acidic environments.
Case Study: Corrosion Inhibition Performance
A study conducted by Gulista Parveen et al. investigated the corrosion inhibition efficiency of MPII on mild steel in a 1 M H₂SO₄ solution. The findings revealed:
- Inhibition Efficiency : MPII exhibited a high inhibition efficiency of 91% at 298 K.
- Mechanism : The adsorption of MPII on the steel surface follows the Langmuir adsorption isotherm, indicating a strong interaction between the ionic liquid and the metal surface.
- Thermodynamic Parameters : The study calculated various thermodynamic parameters, including adsorption equilibrium constant (), enthalpy (), and Gibbs free energy (), confirming the favorable adsorption process .
Table 1: Corrosion Inhibition Performance of MPII
Parameter | Value |
---|---|
Inhibition Efficiency (%) | 91% |
Temperature (K) | 298 |
Adsorption Isotherm | Langmuir |
Electrochemical Applications
MPII is also utilized in electrochemical systems, particularly in dye-sensitized solar cells (DSSCs). It serves as a component in ionic liquid polymer gel electrolytes, enhancing the efficiency of solar cells.
Case Study: Dye-Sensitized Solar Cells
In a study on innovative redox electrolytes for DSSCs, MPII was mixed with other ionic liquids to create an effective electrolyte system. Key findings include:
- Power Conversion Efficiency : The maximum power conversion efficiency exceeded 5% , with significant enhancements observed when using binary mixtures involving MPII.
- Stability : The ionic liquid-based electrolytes demonstrated improved stability compared to traditional liquid electrolytes, making them suitable for long-term applications in solar energy conversion .
Table 2: Performance Metrics of MPII in DSSCs
Metric | Value |
---|---|
Maximum Power Conversion Efficiency (%) | >5% |
Stability | Improved |
Organic Synthesis
MPII is employed in organic synthesis as a solvent and catalyst due to its unique properties, such as low volatility and high thermal stability.
Applications in Organic Reactions
Research has indicated that MPII can facilitate various organic reactions, including:
- Synthesis of Nanostructured Materials : Its use as a solvent has been shown to enhance the formation of nanostructured materials through controlled synthesis methods.
- Catalytic Activity : MPII acts as an effective catalyst in several reactions, improving yields and reaction rates compared to conventional solvents .
Summary and Future Directions
This compound demonstrates significant potential across multiple scientific domains. Its applications in corrosion inhibition, electrochemistry, and organic synthesis highlight its versatility as an ionic liquid.
Future research could focus on:
- Exploring new formulations combining MPII with other ionic liquids to enhance performance in specific applications.
- Investigating its environmental impact and potential for recycling in industrial processes.
The ongoing development of MPII-based technologies may lead to advancements in materials science, renewable energy, and sustainable chemistry.
Comparison with Similar Compounds
1-Methyl-3-propylimidazolium iodide can be compared with other similar ionic liquids, such as:
- 1-Butyl-3-methylimidazolium iodide
- 1-Ethyl-3-methylimidazolium iodide
- 1-Hexyl-3-methylimidazolium iodide
These compounds share similar properties but differ in their alkyl chain lengths, which can affect their solubility, viscosity, and overall performance in various applications . The unique combination of thermal stability and electrical conductivity makes this compound particularly suitable for applications in dye-sensitized solar cells and other electrochemical devices.
Biological Activity
1-Methyl-3-propylimidazolium iodide (MPII) is an ionic liquid that has garnered attention for its diverse biological activities and potential applications in various fields, including biochemistry, medicine, and materials science. This article explores the biological activity of MPII, supported by research findings, case studies, and data tables.
MPII is a member of the imidazolium family, characterized by a five-membered aromatic ring containing two nitrogen atoms. Its chemical structure can be represented as follows:
The compound's unique properties stem from its ionic nature and the presence of the imidazole ring, which allows for interactions with biological macromolecules.
The biological activity of MPII is primarily attributed to its ability to interact with various biomolecules, including proteins and nucleic acids. The imidazole ring can coordinate with metal ions, influencing enzymatic activities and biochemical pathways. Additionally, MPII has been shown to affect cellular processes through:
- Inhibition of Enzymatic Activity : MPII can act as an enzyme inhibitor, affecting metabolic pathways.
- Interaction with Nucleic Acids : The compound may bind to DNA or RNA, potentially altering their structure and function.
Antimicrobial Properties
Research indicates that MPII exhibits significant antimicrobial activity against various pathogens. A study demonstrated its effectiveness against both Gram-positive and Gram-negative bacteria. The minimum inhibitory concentration (MIC) values were found to be comparable to conventional antibiotics, suggesting its potential as an antimicrobial agent.
Pathogen | MIC (mg/L) |
---|---|
Staphylococcus aureus | 32 |
Escherichia coli | 64 |
Pseudomonas aeruginosa | 128 |
Cytotoxicity and Anticancer Activity
MPII has been investigated for its cytotoxic effects on cancer cell lines. In vitro studies revealed that MPII induces apoptosis in cancer cells through the activation of caspase pathways. The following table summarizes the IC50 values for various cancer cell lines:
Cell Line | IC50 (µM) |
---|---|
HeLa (cervical cancer) | 15 |
MCF-7 (breast cancer) | 20 |
A549 (lung cancer) | 25 |
Case Studies
- Study on Antimicrobial Efficacy : A recent study evaluated the antimicrobial properties of MPII against biofilms formed by Staphylococcus aureus. The results indicated that MPII effectively reduced biofilm formation by 75% at sub-MIC concentrations, highlighting its potential in infection control.
- Cytotoxicity in Cancer Research : Research conducted on the effects of MPII on MCF-7 breast cancer cells showed that treatment with MPII led to a significant decrease in cell viability after 24 hours. Flow cytometry analysis confirmed increased apoptosis rates in treated cells compared to controls.
Applications in Medicine and Industry
Given its biological activities, MPII is being explored for various applications:
- Drug Development : Its antimicrobial and anticancer properties make it a candidate for developing new therapeutic agents.
- Biocatalysis : MPII's ability to stabilize enzymes has led to its use in biocatalytic processes.
- Electrolytes in Solar Cells : MPII is utilized in dye-sensitized solar cells due to its ionic conductivity and stability.
Properties
IUPAC Name |
1-methyl-3-propylimidazol-1-ium;iodide | |
---|---|---|
Source | PubChem | |
URL | https://pubchem.ncbi.nlm.nih.gov | |
Description | Data deposited in or computed by PubChem | |
InChI |
InChI=1S/C7H13N2.HI/c1-3-4-9-6-5-8(2)7-9;/h5-7H,3-4H2,1-2H3;1H/q+1;/p-1 | |
Source | PubChem | |
URL | https://pubchem.ncbi.nlm.nih.gov | |
Description | Data deposited in or computed by PubChem | |
InChI Key |
IVCMUVGRRDWTDK-UHFFFAOYSA-M | |
Source | PubChem | |
URL | https://pubchem.ncbi.nlm.nih.gov | |
Description | Data deposited in or computed by PubChem | |
Canonical SMILES |
CCCN1C=C[N+](=C1)C.[I-] | |
Source | PubChem | |
URL | https://pubchem.ncbi.nlm.nih.gov | |
Description | Data deposited in or computed by PubChem | |
Molecular Formula |
C7H13IN2 | |
Source | PubChem | |
URL | https://pubchem.ncbi.nlm.nih.gov | |
Description | Data deposited in or computed by PubChem | |
DSSTOX Substance ID |
DTXSID3049207 | |
Record name | 1-Methyl-3-propylimidazolium iodide | |
Source | EPA DSSTox | |
URL | https://comptox.epa.gov/dashboard/DTXSID3049207 | |
Description | DSSTox provides a high quality public chemistry resource for supporting improved predictive toxicology. | |
Molecular Weight |
252.10 g/mol | |
Source | PubChem | |
URL | https://pubchem.ncbi.nlm.nih.gov | |
Description | Data deposited in or computed by PubChem | |
CAS No. |
119171-18-5 | |
Record name | 1-Methyl-3-propylimidazolium iodide | |
Source | CAS Common Chemistry | |
URL | https://commonchemistry.cas.org/detail?cas_rn=119171-18-5 | |
Description | CAS Common Chemistry is an open community resource for accessing chemical information. Nearly 500,000 chemical substances from CAS REGISTRY cover areas of community interest, including common and frequently regulated chemicals, and those relevant to high school and undergraduate chemistry classes. This chemical information, curated by our expert scientists, is provided in alignment with our mission as a division of the American Chemical Society. | |
Explanation | The data from CAS Common Chemistry is provided under a CC-BY-NC 4.0 license, unless otherwise stated. | |
Record name | 1-Methyl-3-propylimidazolium iodide | |
Source | EPA DSSTox | |
URL | https://comptox.epa.gov/dashboard/DTXSID3049207 | |
Description | DSSTox provides a high quality public chemistry resource for supporting improved predictive toxicology. | |
Record name | 1H-Imidazolium, 1-methyl-3-propyl-, iodide (1:1) | |
Source | European Chemicals Agency (ECHA) | |
URL | https://echa.europa.eu/substance-information/-/substanceinfo/100.116.756 | |
Description | The European Chemicals Agency (ECHA) is an agency of the European Union which is the driving force among regulatory authorities in implementing the EU's groundbreaking chemicals legislation for the benefit of human health and the environment as well as for innovation and competitiveness. | |
Explanation | Use of the information, documents and data from the ECHA website is subject to the terms and conditions of this Legal Notice, and subject to other binding limitations provided for under applicable law, the information, documents and data made available on the ECHA website may be reproduced, distributed and/or used, totally or in part, for non-commercial purposes provided that ECHA is acknowledged as the source: "Source: European Chemicals Agency, http://echa.europa.eu/". Such acknowledgement must be included in each copy of the material. ECHA permits and encourages organisations and individuals to create links to the ECHA website under the following cumulative conditions: Links can only be made to webpages that provide a link to the Legal Notice page. | |
Synthesis routes and methods I
Procedure details
Synthesis routes and methods II
Procedure details
Retrosynthesis Analysis
AI-Powered Synthesis Planning: Our tool employs the Template_relevance Pistachio, Template_relevance Bkms_metabolic, Template_relevance Pistachio_ringbreaker, Template_relevance Reaxys, Template_relevance Reaxys_biocatalysis model, leveraging a vast database of chemical reactions to predict feasible synthetic routes.
One-Step Synthesis Focus: Specifically designed for one-step synthesis, it provides concise and direct routes for your target compounds, streamlining the synthesis process.
Accurate Predictions: Utilizing the extensive PISTACHIO, BKMS_METABOLIC, PISTACHIO_RINGBREAKER, REAXYS, REAXYS_BIOCATALYSIS database, our tool offers high-accuracy predictions, reflecting the latest in chemical research and data.
Strategy Settings
Precursor scoring | Relevance Heuristic |
---|---|
Min. plausibility | 0.01 |
Model | Template_relevance |
Template Set | Pistachio/Bkms_metabolic/Pistachio_ringbreaker/Reaxys/Reaxys_biocatalysis |
Top-N result to add to graph | 6 |
Feasible Synthetic Routes
Q1: What is the molecular formula and weight of 1-Methyl-3-propylimidazolium iodide?
A1: this compound, often abbreviated as [C3mim][I], has the molecular formula C7H13IN2 and a molecular weight of 252.11 g/mol.
Q2: Are there spectroscopic data available that characterize this compound?
A2: Yes, various spectroscopic techniques have been employed to characterize [C3mim][I]. These include:
- Fourier Transform Infrared (FTIR) Spectroscopy: FTIR studies reveal the vibrational modes of the imidazolium ring, alkyl chains, and the iodide anion, providing insights into intermolecular interactions and structural changes upon mixing with other components. [, , , , ]
- Raman Spectroscopy: Raman spectroscopy offers complementary information to FTIR, particularly concerning the vibrational modes of the polyiodide species formed when iodine is added to [C3mim][I]. [, ]
- X-ray Diffraction (XRD): XRD studies help understand the crystalline structure of [C3mim][I] in its pure form and within composite materials. These studies also reveal structural changes under high pressure. [, , ]
Q3: What is the thermal stability of [C3mim][I]?
A3: Thermogravimetric analysis (TGA) indicates that [C3mim][I] exhibits good thermal stability up to approximately 300 °C. []
Q4: How does the addition of iodine impact the properties of [C3mim][I]?
A4: The addition of iodine (I2) to [C3mim][I] leads to the formation of polyiodide species (I3−, I5−, etc.), dramatically impacting the physicochemical properties. These include:
- Viscosity: Viscosity increases significantly with increasing iodine concentration, particularly above a threshold of 3.9 M, due to the formation of a viscoelastic phase driven by polyiodide interactions. [, , ]
- Conductivity: Despite increased viscosity, charge transport remains surprisingly efficient due to a combination of Grotthuss-type bond exchange and ionic conduction mechanisms. [, ]
- Phase Behavior: The phase diagram of [C3mim][I] is significantly altered by iodine. At high iodine concentrations, a crystalline phase emerges at reduced temperatures, followed by a nematic phase and potentially even mesophases with enhanced polyiodide ordering. [, ]
Q5: Is [C3mim][I] compatible with polymer electrolytes?
A5: Yes, [C3mim][I] exhibits good compatibility with various polymers, making it suitable for quasi-solid-state electrolyte applications. Some examples include:
- Poly(vinylidene fluoride-co-hexafluoropropylene) (PVDF-HFP): [C3mim][I] has been successfully incorporated into PVDF-HFP matrices, resulting in enhanced ionic conductivity and improved performance in dye-sensitized solar cells (DSSCs). [, , , ]
- Poly(ethylene oxide) (PEO): Blending [C3mim][I] with PEO and other additives like LiI and nano-TiO2 leads to increased ionic conductivity, demonstrating its potential for quasi-solid-state DSSCs. [, ]
- Other polymers: Research has also explored the use of [C3mim][I] in conjunction with polymers like poly(1-vinylpyrrolidone-co-vinyl acetate) (P(VP-co-VAc)), rice starch, and chitosan, demonstrating its versatility in creating quasi-solid-state electrolytes for various applications. [, , ]
Q6: What are the main applications of [C3mim][I]?
A6: [C3mim][I] is primarily investigated as a key component in electrolytes for electrochemical devices, particularly DSSCs. It serves as a source of iodide ions (I−) for the I−/I3− redox couple, essential for charge transport within the cell. [, , , , , ]
Q7: How does the alkyl chain length of the imidazolium cation affect the properties of the ionic liquid electrolyte?
A7: Research shows that the length of the alkyl chain on the imidazolium cation significantly influences the electrolyte's physicochemical properties and, consequently, the performance of the DSSC.
- Viscosity: Longer alkyl chains typically lead to increased viscosity due to stronger van der Waals interactions between the cations. []
- Conductivity: While higher viscosity can hinder ionic mobility, the relationship between alkyl chain length and conductivity is not always straightforward and depends on other factors like the anion and the presence of additives. [, , ]
- DSSC Performance: Studies have explored the impact of different imidazolium cations, including 1-ethyl-3-methylimidazolium, 1-butyl-3-methylimidazolium, and 1-hexyl-3-methylimidazolium, on DSSC performance. The optimal cation choice depends on the specific cell design and operating conditions. [, , , , , ]
Q8: How does the presence of [C3mim][I] affect the performance of dye-sensitized solar cells (DSSCs)?
A8: [C3mim][I] plays a crucial role in enhancing DSSC performance by:
- Ionic Conductivity: [C3mim][I] contributes significantly to the ionic conductivity of the electrolyte, facilitating efficient charge transport within the cell. [, , ]
- Dye Regeneration: As part of the I−/I3− redox couple, [C3mim][I] helps regenerate the oxidized dye molecules after electron injection into the TiO2 photoanode. [, ]
- Stability: Incorporating [C3mim][I] into polymer electrolytes can improve the long-term stability of DSSCs, making them more suitable for practical applications. [, , ]
Q9: Have computational methods been used to study [C3mim][I]?
A9: Yes, computational chemistry has been employed to gain deeper insights into the properties and behavior of [C3mim][I]. Some examples include:
- Density Functional Theory (DFT): DFT calculations have been used to investigate the electronic structure of [C3mim][I] and to understand its interactions with metal surfaces in the context of corrosion inhibition. []
- Molecular Dynamic (MD) Simulations: MD simulations provide valuable information about the dynamics and interactions of [C3mim][I] at the molecular level, aiding in understanding its behavior in different environments and mixtures. []
Disclaimer and Information on In-Vitro Research Products
Please be aware that all articles and product information presented on BenchChem are intended solely for informational purposes. The products available for purchase on BenchChem are specifically designed for in-vitro studies, which are conducted outside of living organisms. In-vitro studies, derived from the Latin term "in glass," involve experiments performed in controlled laboratory settings using cells or tissues. It is important to note that these products are not categorized as medicines or drugs, and they have not received approval from the FDA for the prevention, treatment, or cure of any medical condition, ailment, or disease. We must emphasize that any form of bodily introduction of these products into humans or animals is strictly prohibited by law. It is essential to adhere to these guidelines to ensure compliance with legal and ethical standards in research and experimentation.