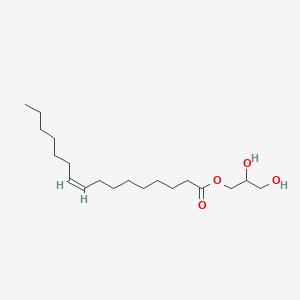
Monopalmitolein
Overview
Description
1-[(9Z)-hexadecenoyl]glycerol is a 1-monoglyceride in which the acyl group is specified as (9Z)-hexadecenoyl. It is functionally related to a palmitoleic acid.
Mechanism of Action
Target of Action
Monopalmitolein, also known as 1-Palmitoleoyl glycerol, is a biologically active monoacylglycerol . It is primarily used in the crystallization of membrane proteins with large extracellular domains . The primary targets of this compound are these membrane proteins.
Mode of Action
This compound interacts with its targets, the membrane proteins, by creating thermodynamically stable ultraswollen bicontinuous cubic phases with water channels . These cubic phases are five times larger than traditional lipidic mesophases, making them suitable for the crystallization of membrane proteins with large extracellular domains .
Biochemical Pathways
It is known that this compound plays a crucial role in the crystallization of membrane proteins, which are integral to various cellular processes and biochemical pathways .
Pharmacokinetics
It is known to be soluble in hexane , which may influence its bioavailability.
Result of Action
This compound’s action results in the formation of stable cubic phases suitable for the crystallization of membrane proteins . This can facilitate the study of these proteins’ structures and functions, contributing to our understanding of various biological processes and diseases.
Action Environment
The action of this compound is influenced by environmental factors such as temperature and the presence of other compounds. For instance, it is stored at -20°C to maintain its stability . Furthermore, it is used in combination with DSPG (Distearoylphosphatidylglycerol) to create the cubic phases necessary for protein crystallization .
Biochemical Analysis
Biochemical Properties
Monopalmitolein plays a significant role in biochemical reactions. It interacts with various enzymes, proteins, and other biomolecules. For instance, it has been shown to inhibit P-glycoprotein in Caco-2 cells .
Cellular Effects
This compound influences cell function in several ways. It has been observed to increase daunomycin accumulation in cells . This compound also induces thymocyte apoptosis, affecting cellular metabolism and gene expression .
Molecular Mechanism
At the molecular level, this compound exerts its effects through various mechanisms. It binds to biomolecules, inhibits or activates enzymes, and alters gene expression. For example, it inhibits P-glycoprotein, which can affect the transport of various substances across the cell membrane .
Properties
IUPAC Name |
2,3-dihydroxypropyl (Z)-hexadec-9-enoate | |
---|---|---|
Source | PubChem | |
URL | https://pubchem.ncbi.nlm.nih.gov | |
Description | Data deposited in or computed by PubChem | |
InChI |
InChI=1S/C19H36O4/c1-2-3-4-5-6-7-8-9-10-11-12-13-14-15-19(22)23-17-18(21)16-20/h7-8,18,20-21H,2-6,9-17H2,1H3/b8-7- | |
Source | PubChem | |
URL | https://pubchem.ncbi.nlm.nih.gov | |
Description | Data deposited in or computed by PubChem | |
InChI Key |
KVYUBFKSKZWZSV-FPLPWBNLSA-N | |
Source | PubChem | |
URL | https://pubchem.ncbi.nlm.nih.gov | |
Description | Data deposited in or computed by PubChem | |
Canonical SMILES |
CCCCCCC=CCCCCCCCC(=O)OCC(CO)O | |
Source | PubChem | |
URL | https://pubchem.ncbi.nlm.nih.gov | |
Description | Data deposited in or computed by PubChem | |
Isomeric SMILES |
CCCCCC/C=C\CCCCCCCC(=O)OCC(CO)O | |
Source | PubChem | |
URL | https://pubchem.ncbi.nlm.nih.gov | |
Description | Data deposited in or computed by PubChem | |
Molecular Formula |
C19H36O4 | |
Source | PubChem | |
URL | https://pubchem.ncbi.nlm.nih.gov | |
Description | Data deposited in or computed by PubChem | |
DSSTOX Substance ID |
DTXSID601316256 | |
Record name | 1-Monopalmitolein | |
Source | EPA DSSTox | |
URL | https://comptox.epa.gov/dashboard/DTXSID601316256 | |
Description | DSSTox provides a high quality public chemistry resource for supporting improved predictive toxicology. | |
Molecular Weight |
328.5 g/mol | |
Source | PubChem | |
URL | https://pubchem.ncbi.nlm.nih.gov | |
Description | Data deposited in or computed by PubChem | |
CAS No. |
37515-61-0 | |
Record name | 1-Monopalmitolein | |
Source | CAS Common Chemistry | |
URL | https://commonchemistry.cas.org/detail?cas_rn=37515-61-0 | |
Description | CAS Common Chemistry is an open community resource for accessing chemical information. Nearly 500,000 chemical substances from CAS REGISTRY cover areas of community interest, including common and frequently regulated chemicals, and those relevant to high school and undergraduate chemistry classes. This chemical information, curated by our expert scientists, is provided in alignment with our mission as a division of the American Chemical Society. | |
Explanation | The data from CAS Common Chemistry is provided under a CC-BY-NC 4.0 license, unless otherwise stated. | |
Record name | 1-Monopalmitolein | |
Source | EPA DSSTox | |
URL | https://comptox.epa.gov/dashboard/DTXSID601316256 | |
Description | DSSTox provides a high quality public chemistry resource for supporting improved predictive toxicology. | |
Retrosynthesis Analysis
AI-Powered Synthesis Planning: Our tool employs the Template_relevance Pistachio, Template_relevance Bkms_metabolic, Template_relevance Pistachio_ringbreaker, Template_relevance Reaxys, Template_relevance Reaxys_biocatalysis model, leveraging a vast database of chemical reactions to predict feasible synthetic routes.
One-Step Synthesis Focus: Specifically designed for one-step synthesis, it provides concise and direct routes for your target compounds, streamlining the synthesis process.
Accurate Predictions: Utilizing the extensive PISTACHIO, BKMS_METABOLIC, PISTACHIO_RINGBREAKER, REAXYS, REAXYS_BIOCATALYSIS database, our tool offers high-accuracy predictions, reflecting the latest in chemical research and data.
Strategy Settings
Precursor scoring | Relevance Heuristic |
---|---|
Min. plausibility | 0.01 |
Model | Template_relevance |
Template Set | Pistachio/Bkms_metabolic/Pistachio_ringbreaker/Reaxys/Reaxys_biocatalysis |
Top-N result to add to graph | 6 |
Feasible Synthetic Routes
Q1: What are the antifungal properties of monopalmitolein and how do they relate to White-Nose Syndrome in bats?
A1: this compound, along with other fatty acid esters like 1-monoolein, 1-monolinolein, behenyl oleate, and behenyl palmitoleate, have demonstrated significant antifungal activity against Pseudogymnoascus destructans (Pd) []. This fungus is the causative agent of White-Nose Syndrome (WNS), a devastating disease affecting North American bat populations. Interestingly, bat species resistant to Pd infections have been found to have these specific fatty acid esters present in their epidermis or sebum [], suggesting a role for these compounds in natural defenses against WNS. Importantly, the study demonstrated that these antifungal fatty acid esters are not hydrolyzed by the lipases secreted by Pd, unlike esters that do not inhibit fungal growth [].
Q2: How does this compound influence the structure of lyotropic liquid crystalline lipid nanoparticles?
A2: this compound, alongside monoolein and phytantriol, can be incorporated into lipid nanoparticles, impacting their internal structure []. The presence of saturated fatty acids (FAs) alongside this compound in these nanoparticles induces a shift towards structures with higher negative surface curvatures as temperature increases. This shift progresses from inverse bicontinuous cubic phases to hexagonal phases, ultimately leading to emulsified microemulsions []. The specific fatty acid chain length and concentration further influence the nanoparticle's internal mesophase structure and lattice parameters [].
Q3: Can this compound be used in the crystallization of membrane proteins, and if so, how does it compare to other lipids?
A3: Yes, this compound, similar to monoolein, can form bicontinuous cubic phases suitable for the crystallization of membrane proteins [, ]. These phases can accommodate a large amount of water (up to 80 wt%), making them ideal for crystallizing membrane proteins with large extracellular domains []. While monoolein is more commonly used, this compound offers an alternative, and the choice of lipid might depend on the specific protein and crystallization conditions.
Q4: Is this compound compatible with high-throughput screening methods for membrane protein crystallization?
A4: Research indicates that this compound is compatible with at least one commercially available high-throughput crystallization screen designed for cubic mesophases, the Cubic crystallization screen []. This screen, primarily composed of poly(ethylene glycol) (PEG) and salt components, was found to be compatible with cubic mesophase retention for this compound under most crystallization conditions tested []. This suggests that this compound could be incorporated into high-throughput workflows for membrane protein crystallization.
Disclaimer and Information on In-Vitro Research Products
Please be aware that all articles and product information presented on BenchChem are intended solely for informational purposes. The products available for purchase on BenchChem are specifically designed for in-vitro studies, which are conducted outside of living organisms. In-vitro studies, derived from the Latin term "in glass," involve experiments performed in controlled laboratory settings using cells or tissues. It is important to note that these products are not categorized as medicines or drugs, and they have not received approval from the FDA for the prevention, treatment, or cure of any medical condition, ailment, or disease. We must emphasize that any form of bodily introduction of these products into humans or animals is strictly prohibited by law. It is essential to adhere to these guidelines to ensure compliance with legal and ethical standards in research and experimentation.