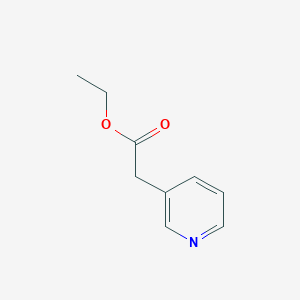
Ethyl 3-pyridylacetate
Overview
Description
Synthesis Analysis
The synthesis of Ethyl 3-pyridylacetate can be achieved by refluxing pyridine 3-acetate and ethanol in the presence of an acid catalyst . Another method involves refluxing 3-acetylchloropyridine first, and then reacting it with absolute ethanol .Molecular Structure Analysis
The molecular structure of Ethyl 3-pyridylacetate can be represented by the SMILES stringCCOC(=O)Cc1cccnc1
. The InChI representation is InChI=1S/C9H11NO2/c1-2-12-9(11)6-8-4-3-5-10-7-8/h3-5,7H,2,6H2,1H3
. Chemical Reactions Analysis
While specific chemical reactions involving Ethyl 3-pyridylacetate are not detailed in the retrieved information, it is known to be a reagent in the preparation of piperidinylalkanoic acid derivatives .Physical And Chemical Properties Analysis
Ethyl 3-pyridylacetate is a clear colorless to yellow liquid . It has a molecular weight of 165.19 . Its density is 1.086 g/mL at 25 °C, and it has a boiling point of 80 °C/0.4 mmHg . The compound is slightly soluble in chloroform and methanol .Scientific Research Applications
Chemical Composition
Ethyl 3-pyridylacetate is an organic compound with the molecular formula C9H11NO2 . It is a clear colorless to yellow liquid .
Chemical Properties
It has a refractive index of 1.4990 to 1.5010 at 20°C, 589 nm . .
Chemical Synthesis
Ethyl 3-pyridylacetate is used as a reagent in the preparation of piperidinylalkanoic acid derivatives . These derivatives are potent α4β1 integrin antagonists .
Biological Applications
The piperidinylalkanoic acid derivatives, synthesized using Ethyl 3-pyridylacetate, act as potent α4β1 integrin antagonists . Integrins are proteins that function as receptors in cells and play a crucial role in cell signaling. Antagonists of these proteins can be used in the treatment of various diseases, including inflammatory diseases and cancer.
Research Use
Ethyl 3-pyridylacetate is marked as RUO – Research Use Only . This indicates that it is primarily used in laboratory research settings and not intended for use in diagnostic or therapeutic applications.
Commercial Availability
Ethyl 3-pyridylacetate is commercially available and is sold by various chemical suppliers, including Thermo Scientific Chemicals . It is typically sold in glass bottles in quantities of 25 g or 5 g .
Safety and Hazards
Ethyl 3-pyridylacetate is considered hazardous. It is irritating to eyes, respiratory system, and skin . Safety measures include avoiding breathing its dust/fume/gas/mist/vapours/spray, washing hands thoroughly after handling, and wearing protective equipment . In case of contact with eyes, it is advised to rinse immediately with plenty of water and seek medical advice .
Mechanism of Action
Target of Action
Ethyl 3-pyridylacetate is primarily used as a reagent in the preparation of piperidinylalkanoic acid derivatives, which are potent antagonists of the α4β1 integrin . The α4β1 integrin is a protein that plays a crucial role in cell adhesion and migration, particularly in the immune response and in the development of various tissues .
Mode of Action
It is known to interact with its target, the α4β1 integrin, through the formation of piperidinylalkanoic acid derivatives . These derivatives act as antagonists, inhibiting the function of the α4β1 integrin .
Biochemical Pathways
The biochemical pathways affected by Ethyl 3-pyridylacetate are related to the functions of the α4β1 integrin. By inhibiting this integrin, Ethyl 3-pyridylacetate can affect cell adhesion and migration processes . This can have downstream effects on immune response and tissue development .
Pharmacokinetics
Its physical properties such as its boiling point (80 °c/04 mmHg) and density (1086 g/mL at 25 °C) have been reported .
Result of Action
The molecular and cellular effects of Ethyl 3-pyridylacetate’s action primarily involve the inhibition of the α4β1 integrin. This can lead to changes in cell adhesion and migration, potentially affecting immune response and tissue development .
Action Environment
Like all chemical compounds, its stability and reactivity can be influenced by factors such as temperature, ph, and the presence of other chemicals .
properties
IUPAC Name |
ethyl 2-pyridin-3-ylacetate | |
---|---|---|
Source | PubChem | |
URL | https://pubchem.ncbi.nlm.nih.gov | |
Description | Data deposited in or computed by PubChem | |
InChI |
InChI=1S/C9H11NO2/c1-2-12-9(11)6-8-4-3-5-10-7-8/h3-5,7H,2,6H2,1H3 | |
Source | PubChem | |
URL | https://pubchem.ncbi.nlm.nih.gov | |
Description | Data deposited in or computed by PubChem | |
InChI Key |
RPWXYCRIAGBAGY-UHFFFAOYSA-N | |
Source | PubChem | |
URL | https://pubchem.ncbi.nlm.nih.gov | |
Description | Data deposited in or computed by PubChem | |
Canonical SMILES |
CCOC(=O)CC1=CN=CC=C1 | |
Source | PubChem | |
URL | https://pubchem.ncbi.nlm.nih.gov | |
Description | Data deposited in or computed by PubChem | |
Molecular Formula |
C9H11NO2 | |
Source | PubChem | |
URL | https://pubchem.ncbi.nlm.nih.gov | |
Description | Data deposited in or computed by PubChem | |
DSSTOX Substance ID |
DTXSID50192984 | |
Record name | 3-Pyridineacetic acid, ethyl ester | |
Source | EPA DSSTox | |
URL | https://comptox.epa.gov/dashboard/DTXSID50192984 | |
Description | DSSTox provides a high quality public chemistry resource for supporting improved predictive toxicology. | |
Molecular Weight |
165.19 g/mol | |
Source | PubChem | |
URL | https://pubchem.ncbi.nlm.nih.gov | |
Description | Data deposited in or computed by PubChem | |
Product Name |
Ethyl 3-pyridylacetate | |
CAS RN |
39931-77-6 | |
Record name | 3-Pyridineacetic acid, ethyl ester | |
Source | CAS Common Chemistry | |
URL | https://commonchemistry.cas.org/detail?cas_rn=39931-77-6 | |
Description | CAS Common Chemistry is an open community resource for accessing chemical information. Nearly 500,000 chemical substances from CAS REGISTRY cover areas of community interest, including common and frequently regulated chemicals, and those relevant to high school and undergraduate chemistry classes. This chemical information, curated by our expert scientists, is provided in alignment with our mission as a division of the American Chemical Society. | |
Explanation | The data from CAS Common Chemistry is provided under a CC-BY-NC 4.0 license, unless otherwise stated. | |
Record name | 3-Pyridineacetic acid, ethyl ester | |
Source | ChemIDplus | |
URL | https://pubchem.ncbi.nlm.nih.gov/substance/?source=chemidplus&sourceid=0039931776 | |
Description | ChemIDplus is a free, web search system that provides access to the structure and nomenclature authority files used for the identification of chemical substances cited in National Library of Medicine (NLM) databases, including the TOXNET system. | |
Record name | Ethyl 3-pyridylacetate | |
Source | DTP/NCI | |
URL | https://dtp.cancer.gov/dtpstandard/servlet/dwindex?searchtype=NSC&outputformat=html&searchlist=76091 | |
Description | The NCI Development Therapeutics Program (DTP) provides services and resources to the academic and private-sector research communities worldwide to facilitate the discovery and development of new cancer therapeutic agents. | |
Explanation | Unless otherwise indicated, all text within NCI products is free of copyright and may be reused without our permission. Credit the National Cancer Institute as the source. | |
Record name | 3-Pyridineacetic acid, ethyl ester | |
Source | EPA DSSTox | |
URL | https://comptox.epa.gov/dashboard/DTXSID50192984 | |
Description | DSSTox provides a high quality public chemistry resource for supporting improved predictive toxicology. | |
Record name | Ethyl 3-pyridylacetate | |
Source | European Chemicals Agency (ECHA) | |
URL | https://echa.europa.eu/substance-information/-/substanceinfo/100.049.716 | |
Description | The European Chemicals Agency (ECHA) is an agency of the European Union which is the driving force among regulatory authorities in implementing the EU's groundbreaking chemicals legislation for the benefit of human health and the environment as well as for innovation and competitiveness. | |
Explanation | Use of the information, documents and data from the ECHA website is subject to the terms and conditions of this Legal Notice, and subject to other binding limitations provided for under applicable law, the information, documents and data made available on the ECHA website may be reproduced, distributed and/or used, totally or in part, for non-commercial purposes provided that ECHA is acknowledged as the source: "Source: European Chemicals Agency, http://echa.europa.eu/". Such acknowledgement must be included in each copy of the material. ECHA permits and encourages organisations and individuals to create links to the ECHA website under the following cumulative conditions: Links can only be made to webpages that provide a link to the Legal Notice page. | |
Record name | ETHYL 3-PYRIDYLACETATE | |
Source | FDA Global Substance Registration System (GSRS) | |
URL | https://gsrs.ncats.nih.gov/ginas/app/beta/substances/EA77BEX7VQ | |
Description | The FDA Global Substance Registration System (GSRS) enables the efficient and accurate exchange of information on what substances are in regulated products. Instead of relying on names, which vary across regulatory domains, countries, and regions, the GSRS knowledge base makes it possible for substances to be defined by standardized, scientific descriptions. | |
Explanation | Unless otherwise noted, the contents of the FDA website (www.fda.gov), both text and graphics, are not copyrighted. They are in the public domain and may be republished, reprinted and otherwise used freely by anyone without the need to obtain permission from FDA. Credit to the U.S. Food and Drug Administration as the source is appreciated but not required. | |
Synthesis routes and methods
Procedure details
Retrosynthesis Analysis
AI-Powered Synthesis Planning: Our tool employs the Template_relevance Pistachio, Template_relevance Bkms_metabolic, Template_relevance Pistachio_ringbreaker, Template_relevance Reaxys, Template_relevance Reaxys_biocatalysis model, leveraging a vast database of chemical reactions to predict feasible synthetic routes.
One-Step Synthesis Focus: Specifically designed for one-step synthesis, it provides concise and direct routes for your target compounds, streamlining the synthesis process.
Accurate Predictions: Utilizing the extensive PISTACHIO, BKMS_METABOLIC, PISTACHIO_RINGBREAKER, REAXYS, REAXYS_BIOCATALYSIS database, our tool offers high-accuracy predictions, reflecting the latest in chemical research and data.
Strategy Settings
Precursor scoring | Relevance Heuristic |
---|---|
Min. plausibility | 0.01 |
Model | Template_relevance |
Template Set | Pistachio/Bkms_metabolic/Pistachio_ringbreaker/Reaxys/Reaxys_biocatalysis |
Top-N result to add to graph | 6 |
Feasible Synthetic Routes
Q & A
Q1: What makes Ethyl 3-pyridylacetate useful in organic synthesis?
A1: Ethyl 3-pyridylacetate possesses an active methylene group, making it susceptible to reactions with various electrophiles. [, , ] This allows for the introduction of diverse substituents at the alpha position, broadening its synthetic utility. For instance, it reacts with carbon disulfide under basic conditions, leading to ketene dithioacetals, which can be further transformed into substituted thiophenes. []
Q2: Can you provide an example of Ethyl 3-pyridylacetate's application in medicinal chemistry?
A2: Certainly! Ethyl 3-pyridylacetate plays a key role in synthesizing potent and selective PDE4 inhibitors like L-869,298. [] The lithium enolate of ethyl 3-pyridylacetate N-oxide undergoes an intriguing displacement reaction with a chiral aryl-heteroaryl secondary tosylate. This reaction proceeds with inversion of configuration, offering a route to synthesize chiral trisubstituted methanes, essential building blocks for the target inhibitor. []
Q3: Are there any reported transformations of Ethyl 3-pyridylacetate leading to heterocyclic systems?
A3: Yes, researchers have successfully utilized Ethyl 3-pyridylacetate in the synthesis of 3-vinylpiperidine. [] This highlights the potential of Ethyl 3-pyridylacetate as a starting material for accessing diverse heterocyclic scaffolds, which hold significant importance in medicinal chemistry.
Q4: Can you elaborate on the reaction of Ethyl 3-pyridylacetate with ethyl phenylpropiolate?
A4: Under basic conditions, Ethyl 3-pyridylacetate condenses with ethyl phenylpropiolate to yield ethyl 3,5-diphenyl-4-carbethoxy-6-(3-pyridyl)salicylate. [] This reaction exemplifies the compound's ability to participate in condensation reactions, leading to highly substituted aromatic compounds with potential biological activity.
Disclaimer and Information on In-Vitro Research Products
Please be aware that all articles and product information presented on BenchChem are intended solely for informational purposes. The products available for purchase on BenchChem are specifically designed for in-vitro studies, which are conducted outside of living organisms. In-vitro studies, derived from the Latin term "in glass," involve experiments performed in controlled laboratory settings using cells or tissues. It is important to note that these products are not categorized as medicines or drugs, and they have not received approval from the FDA for the prevention, treatment, or cure of any medical condition, ailment, or disease. We must emphasize that any form of bodily introduction of these products into humans or animals is strictly prohibited by law. It is essential to adhere to these guidelines to ensure compliance with legal and ethical standards in research and experimentation.