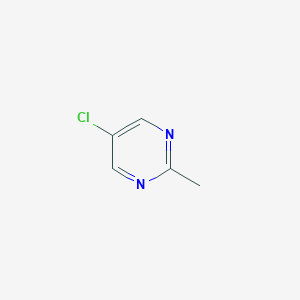
5-Chloro-2-methylpyrimidine
Overview
Description
5-Chloro-2-methylpyrimidine is used in organic synthesis, as a catalytic agent, petrochemical additive, and synthetic chemistry . It is also used as an intermediate for pharmaceuticals .
Molecular Structure Analysis
The molecular formula of 5-Chloro-2-methylpyrimidine is C5H5ClN2 . Its molecular weight is 128.56 .Physical And Chemical Properties Analysis
The physical and chemical properties of 5-Chloro-2-methylpyrimidine include a melting point of 56-57 °C, a predicted boiling point of 169.3±13.0 °C, and a predicted density of 1.234±0.06 g/cm3 . It is stored in a dry, sealed container at room temperature .Scientific Research Applications
Organic Synthesis
5-Chloro-2-methylpyrimidine is used in organic synthesis . Organic synthesis is a method of preparation of organic compounds. It is a science based on a set of principles and concepts that govern the design of synthetic routes, including the selection of reagents and reaction conditions.
Catalytic Agent
This compound serves as a catalytic agent in various chemical reactions . A catalyst is a substance that can increase the rate of a reaction by lowering the activation energy, but remains chemically unchanged after the reaction.
Petrochemical Additive
5-Chloro-2-methylpyrimidine is used as an additive in the petrochemical industry . Additives are substances formulated for improvement of the anti-friction, chemical and physical properties of base oils (mineral, synthetic, vegetable or animal), which results in enhancing the lubricant performance and extending the equipment life.
Synthetic Chemistry
It is also used in synthetic chemistry . Synthetic chemistry involves the creation of new compounds from existing ones and the study of how they react.
Pharmaceutical Intermediate
5-Chloro-2-methylpyrimidine is used as an intermediate for pharmaceuticals . An intermediate is a substance produced during the middle stages of a reaction between two or more other chemicals. Many pharmaceuticals are produced through complex reactions that involve several steps, each requiring specific reagents and conditions.
Material Science
In the field of material science , 5-Chloro-2-methylpyrimidine can be used in the synthesis of new materials with potential applications in various industries .
Safety and Hazards
5-Chloro-2-methylpyrimidine is classified as a hazardous substance. It is a highly flammable liquid and vapor. Precautionary measures include keeping away from heat, sparks, open flames, and hot surfaces, and avoiding smoking. The container should be kept tightly closed, and protective gloves, eye protection, and face protection should be worn .
Future Directions
properties
IUPAC Name |
5-chloro-2-methylpyrimidine | |
---|---|---|
Source | PubChem | |
URL | https://pubchem.ncbi.nlm.nih.gov | |
Description | Data deposited in or computed by PubChem | |
InChI |
InChI=1S/C5H5ClN2/c1-4-7-2-5(6)3-8-4/h2-3H,1H3 | |
Source | PubChem | |
URL | https://pubchem.ncbi.nlm.nih.gov | |
Description | Data deposited in or computed by PubChem | |
InChI Key |
UISREOKYJBBYSC-UHFFFAOYSA-N | |
Source | PubChem | |
URL | https://pubchem.ncbi.nlm.nih.gov | |
Description | Data deposited in or computed by PubChem | |
Canonical SMILES |
CC1=NC=C(C=N1)Cl | |
Source | PubChem | |
URL | https://pubchem.ncbi.nlm.nih.gov | |
Description | Data deposited in or computed by PubChem | |
Molecular Formula |
C5H5ClN2 | |
Source | PubChem | |
URL | https://pubchem.ncbi.nlm.nih.gov | |
Description | Data deposited in or computed by PubChem | |
DSSTOX Substance ID |
DTXSID80304311 | |
Record name | 5-chloro-2-methylpyrimidine | |
Source | EPA DSSTox | |
URL | https://comptox.epa.gov/dashboard/DTXSID80304311 | |
Description | DSSTox provides a high quality public chemistry resource for supporting improved predictive toxicology. | |
Molecular Weight |
128.56 g/mol | |
Source | PubChem | |
URL | https://pubchem.ncbi.nlm.nih.gov | |
Description | Data deposited in or computed by PubChem | |
Product Name |
5-Chloro-2-methylpyrimidine | |
CAS RN |
54198-89-9 | |
Record name | 54198-89-9 | |
Source | DTP/NCI | |
URL | https://dtp.cancer.gov/dtpstandard/servlet/dwindex?searchtype=NSC&outputformat=html&searchlist=165372 | |
Description | The NCI Development Therapeutics Program (DTP) provides services and resources to the academic and private-sector research communities worldwide to facilitate the discovery and development of new cancer therapeutic agents. | |
Explanation | Unless otherwise indicated, all text within NCI products is free of copyright and may be reused without our permission. Credit the National Cancer Institute as the source. | |
Record name | 5-chloro-2-methylpyrimidine | |
Source | EPA DSSTox | |
URL | https://comptox.epa.gov/dashboard/DTXSID80304311 | |
Description | DSSTox provides a high quality public chemistry resource for supporting improved predictive toxicology. | |
Retrosynthesis Analysis
AI-Powered Synthesis Planning: Our tool employs the Template_relevance Pistachio, Template_relevance Bkms_metabolic, Template_relevance Pistachio_ringbreaker, Template_relevance Reaxys, Template_relevance Reaxys_biocatalysis model, leveraging a vast database of chemical reactions to predict feasible synthetic routes.
One-Step Synthesis Focus: Specifically designed for one-step synthesis, it provides concise and direct routes for your target compounds, streamlining the synthesis process.
Accurate Predictions: Utilizing the extensive PISTACHIO, BKMS_METABOLIC, PISTACHIO_RINGBREAKER, REAXYS, REAXYS_BIOCATALYSIS database, our tool offers high-accuracy predictions, reflecting the latest in chemical research and data.
Strategy Settings
Precursor scoring | Relevance Heuristic |
---|---|
Min. plausibility | 0.01 |
Model | Template_relevance |
Template Set | Pistachio/Bkms_metabolic/Pistachio_ringbreaker/Reaxys/Reaxys_biocatalysis |
Top-N result to add to graph | 6 |
Feasible Synthetic Routes
Disclaimer and Information on In-Vitro Research Products
Please be aware that all articles and product information presented on BenchChem are intended solely for informational purposes. The products available for purchase on BenchChem are specifically designed for in-vitro studies, which are conducted outside of living organisms. In-vitro studies, derived from the Latin term "in glass," involve experiments performed in controlled laboratory settings using cells or tissues. It is important to note that these products are not categorized as medicines or drugs, and they have not received approval from the FDA for the prevention, treatment, or cure of any medical condition, ailment, or disease. We must emphasize that any form of bodily introduction of these products into humans or animals is strictly prohibited by law. It is essential to adhere to these guidelines to ensure compliance with legal and ethical standards in research and experimentation.