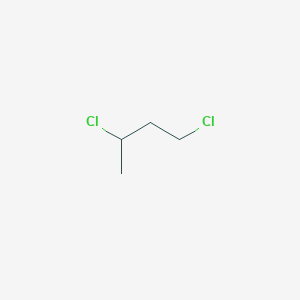
1,3-Dichlorobutane
Overview
Description
Synthesis Analysis
1,3-Dichlorobutane's synthesis and structural analysis have been subjects of study, aiming to understand its formation and configuration. While specific synthesis pathways for this compound are not detailed in the available literature, related compounds such as 1,1-dichlorobutane and other chlorinated butanes have been synthesized through methods that could potentially be adapted for this compound. These methods often involve chlorination reactions under specific conditions to achieve the desired substitution pattern on the butane molecule (Crowder, 1981).
Molecular Structure Analysis
The molecular structure of this compound is pivotal to its chemical behavior. Vibrational spectroscopy analyses, including infrared (IR) and Raman spectroscopy, have been used to deduce the conformations and structural details of similar dichlorobutanes. These studies reveal that such molecules can adopt multiple conformations due to rotational flexibility around the carbon-carbon bonds, which significantly influences their physical and chemical properties (Crowder & Smith, 1979).
Chemical Reactions and Properties
Chemical reactions involving this compound, such as substitution and elimination, are influenced by its dichloro substitution pattern. These reactions are fundamental for further chemical modifications and applications of this compound. Studies on related chlorinated compounds provide insights into potential reactivities, such as nucleophilic substitution reactions that yield various functionalized products (Kooyman & Wagner, 2010).
Scientific Research Applications
Vibrational Analysis : Studies have analyzed the vibrational properties of 1,3-dichlorobutane using infrared and Raman spectra, which aids in understanding its molecular structure and behavior (Crowder & Smith, 1979).
Electrochemical Behavior : Research on the electrochemical reduction of dihalobutanes, including this compound, at carbon cathodes has explored the variety of products formed under different conditions, which has implications in chemical synthesis and electrochemistry (Pritts & Peters, 1995).
Toxicity and Hazard Assessment : There are studies on the toxicity of this compound, which are crucial for understanding its safety and environmental impact. These studies include genotoxicity tests, repeated-dose toxicity tests, and reproductive/developmental toxicity screening in rats (Igarashi et al., 2020).
Thermochemistry : Research has been conducted on the enthalpies of combustion and formation of 1,3-dichlorobutanes, providing valuable data for understanding their thermochemical properties (Xu-wu et al., 1990).
Halogen Migration in Organic Chemistry : Studies have examined the behavior of dichlorobutanes, including this compound, in reactions with aluminum chloride, which is important for understanding halogen migration in organic compounds (Billups et al., 1970).
Radiolysis : Research on the radiolysis of this compound has investigated the stable products formed when it is gamma-irradiated in an oxygen-free atmosphere, contributing to our understanding of its behavior under radiation (Szymański et al., 1986).
Ion-Transfer Voltammetry : The use of this compound in ion-transfer voltammetry has been studied, providing insights into its utility as an organic solvent in this analytical technique (Katano et al., 2004).
Mechanism of Action
Target of Action
1,3-Dichlorobutane is a synthetic organic compound used primarily in the laboratory setting for the synthesis of other substances .
Mode of Action
This compound can undergo a free-radical chain reaction mechanism to produce different isomers of dichlorobutane . This process involves the halogenation of chlorobutane using sulfuryl chloride . The ratio of the different dichlorobutane isomers produced can be determined by gas chromatography .
Biochemical Pathways
The compound’s role in chemical synthesis suggests that it could potentially influence a wide range of biochemical processes depending on the specific context of its use .
Result of Action
The molecular and cellular effects of this compound’s action are largely dependent on the specific context of its use. In chemical synthesis, it can contribute to the formation of a variety of different compounds .
Safety and Hazards
1,3-Dichlorobutane is a flammable liquid and vapor. It causes skin irritation and serious eye irritation. It may also cause respiratory irritation . Safety measures include avoiding dust formation, avoiding breathing mist, gas or vapors, avoiding contact with skin and eye, using personal protective equipment, wearing chemical impermeable gloves, ensuring adequate ventilation, removing all sources of ignition, evacuating personnel to safe areas, and keeping people away from and upwind of spill/leak .
properties
IUPAC Name |
1,3-dichlorobutane | |
---|---|---|
Source | PubChem | |
URL | https://pubchem.ncbi.nlm.nih.gov | |
Description | Data deposited in or computed by PubChem | |
InChI |
InChI=1S/C4H8Cl2/c1-4(6)2-3-5/h4H,2-3H2,1H3 | |
Source | PubChem | |
URL | https://pubchem.ncbi.nlm.nih.gov | |
Description | Data deposited in or computed by PubChem | |
InChI Key |
QBGVARBIQGHVKR-UHFFFAOYSA-N | |
Source | PubChem | |
URL | https://pubchem.ncbi.nlm.nih.gov | |
Description | Data deposited in or computed by PubChem | |
Canonical SMILES |
CC(CCCl)Cl | |
Source | PubChem | |
URL | https://pubchem.ncbi.nlm.nih.gov | |
Description | Data deposited in or computed by PubChem | |
Molecular Formula |
C4H8Cl2 | |
Source | PubChem | |
URL | https://pubchem.ncbi.nlm.nih.gov | |
Description | Data deposited in or computed by PubChem | |
DSSTOX Substance ID |
DTXSID90870855 | |
Record name | Butane, 1,3-dichloro- | |
Source | EPA DSSTox | |
URL | https://comptox.epa.gov/dashboard/DTXSID90870855 | |
Description | DSSTox provides a high quality public chemistry resource for supporting improved predictive toxicology. | |
Molecular Weight |
127.01 g/mol | |
Source | PubChem | |
URL | https://pubchem.ncbi.nlm.nih.gov | |
Description | Data deposited in or computed by PubChem | |
Physical Description |
Colorless liquid; [Sigma-Aldrich MSDS] | |
Record name | 1,3-Dichlorobutane | |
Source | Haz-Map, Information on Hazardous Chemicals and Occupational Diseases | |
URL | https://haz-map.com/Agents/19248 | |
Description | Haz-Map® is an occupational health database designed for health and safety professionals and for consumers seeking information about the adverse effects of workplace exposures to chemical and biological agents. | |
Explanation | Copyright (c) 2022 Haz-Map(R). All rights reserved. Unless otherwise indicated, all materials from Haz-Map are copyrighted by Haz-Map(R). No part of these materials, either text or image may be used for any purpose other than for personal use. Therefore, reproduction, modification, storage in a retrieval system or retransmission, in any form or by any means, electronic, mechanical or otherwise, for reasons other than personal use, is strictly prohibited without prior written permission. | |
CAS RN |
1190-22-3 | |
Record name | 1,3-Dichlorobutane | |
Source | CAS Common Chemistry | |
URL | https://commonchemistry.cas.org/detail?cas_rn=1190-22-3 | |
Description | CAS Common Chemistry is an open community resource for accessing chemical information. Nearly 500,000 chemical substances from CAS REGISTRY cover areas of community interest, including common and frequently regulated chemicals, and those relevant to high school and undergraduate chemistry classes. This chemical information, curated by our expert scientists, is provided in alignment with our mission as a division of the American Chemical Society. | |
Explanation | The data from CAS Common Chemistry is provided under a CC-BY-NC 4.0 license, unless otherwise stated. | |
Record name | Butane, 1,3-dichloro- | |
Source | ChemIDplus | |
URL | https://pubchem.ncbi.nlm.nih.gov/substance/?source=chemidplus&sourceid=0001190223 | |
Description | ChemIDplus is a free, web search system that provides access to the structure and nomenclature authority files used for the identification of chemical substances cited in National Library of Medicine (NLM) databases, including the TOXNET system. | |
Record name | Butane, 1,3-dichloro- | |
Source | EPA Chemicals under the TSCA | |
URL | https://www.epa.gov/chemicals-under-tsca | |
Description | EPA Chemicals under the Toxic Substances Control Act (TSCA) collection contains information on chemicals and their regulations under TSCA, including non-confidential content from the TSCA Chemical Substance Inventory and Chemical Data Reporting. | |
Record name | Butane, 1,3-dichloro- | |
Source | EPA DSSTox | |
URL | https://comptox.epa.gov/dashboard/DTXSID90870855 | |
Description | DSSTox provides a high quality public chemistry resource for supporting improved predictive toxicology. | |
Record name | 1,3-dichlorobutane | |
Source | European Chemicals Agency (ECHA) | |
URL | https://echa.europa.eu/substance-information/-/substanceinfo/100.013.381 | |
Description | The European Chemicals Agency (ECHA) is an agency of the European Union which is the driving force among regulatory authorities in implementing the EU's groundbreaking chemicals legislation for the benefit of human health and the environment as well as for innovation and competitiveness. | |
Explanation | Use of the information, documents and data from the ECHA website is subject to the terms and conditions of this Legal Notice, and subject to other binding limitations provided for under applicable law, the information, documents and data made available on the ECHA website may be reproduced, distributed and/or used, totally or in part, for non-commercial purposes provided that ECHA is acknowledged as the source: "Source: European Chemicals Agency, http://echa.europa.eu/". Such acknowledgement must be included in each copy of the material. ECHA permits and encourages organisations and individuals to create links to the ECHA website under the following cumulative conditions: Links can only be made to webpages that provide a link to the Legal Notice page. | |
Retrosynthesis Analysis
AI-Powered Synthesis Planning: Our tool employs the Template_relevance Pistachio, Template_relevance Bkms_metabolic, Template_relevance Pistachio_ringbreaker, Template_relevance Reaxys, Template_relevance Reaxys_biocatalysis model, leveraging a vast database of chemical reactions to predict feasible synthetic routes.
One-Step Synthesis Focus: Specifically designed for one-step synthesis, it provides concise and direct routes for your target compounds, streamlining the synthesis process.
Accurate Predictions: Utilizing the extensive PISTACHIO, BKMS_METABOLIC, PISTACHIO_RINGBREAKER, REAXYS, REAXYS_BIOCATALYSIS database, our tool offers high-accuracy predictions, reflecting the latest in chemical research and data.
Strategy Settings
Precursor scoring | Relevance Heuristic |
---|---|
Min. plausibility | 0.01 |
Model | Template_relevance |
Template Set | Pistachio/Bkms_metabolic/Pistachio_ringbreaker/Reaxys/Reaxys_biocatalysis |
Top-N result to add to graph | 6 |
Feasible Synthetic Routes
Q & A
Q1: What are the different conformational structures of 1,3-dichlorobutane?
A: Research using vibrational spectroscopy and molecular mechanics calculations revealed that this compound exhibits rotational isomerism. [, ] This means the molecule can exist in different conformations due to rotation around the carbon-carbon single bonds. While the exact number and identification of all conformers were not definitively determined in the studies, molecular mechanics calculations provided insights into the energies and structures of potential conformers. []
Q2: How does the structure of this compound compare to 1,3-dibromobutane in terms of conformational behavior?
A: Both this compound and 1,3-dibromobutane display rotational isomerism, confirmed through vibrational spectroscopy. [] While they share a similar carbon backbone, the difference in halogen substituents (chlorine vs. bromine) influences their conformational preferences and energies. Molecular mechanics calculations were employed to gain a deeper understanding of these subtle differences in conformational behavior. []
Q3: What happens when 1,4-dichlorobutane is exposed to gamma radiation in an oxygen-free environment?
A: Gamma radiolysis of 1,4-dichlorobutane in the absence of oxygen produces a variety of stable products. In the gas phase, hydrogen chloride (HCl), chloromethane (CH3Cl), 1-chlorobutane, and both 1,3- and 1,4-dichlorobutane isomers are formed. [] The liquid phase yields this compound, 1,1,4-trichlorobutane, 1,2,4-trichlorobutane, and dimers and trimers of the parent 1,4-dichlorobutane molecule. [] This detailed product analysis helps elucidate the mechanisms of radiolytic degradation for 1,4-dichlorobutane.
Q4: Can you elaborate on the method used to identify and quantify the products of 1,4-dichlorobutane radiolysis?
A: Researchers employed a combination of sophisticated techniques to analyze the radiolysis products of 1,4-dichlorobutane. Preparative gas chromatography was utilized to separate the pure products. [] Identification was achieved using Nuclear Magnetic Resonance (NMR) spectroscopy, mass spectrometry, and qualitative gas chromatography. [] Finally, quantification of the identified compounds was carried out using potentiometric analysis and quantitative gas chromatography. [] This multifaceted approach allowed for a comprehensive understanding of the radiolysis process.
Q5: What are the potential applications of silent electrical discharge reactions involving ethylene and hydrogen chloride?
A: Research demonstrated that a silent electrical discharge can initiate reactions between ethylene and hydrogen chloride, producing a range of chlorinated hydrocarbons. [] These products, including chloroethane, 1-chlorobutane, and 1,4-dichlorobutane, have various industrial applications. [] For instance, they serve as intermediates in the synthesis of polymers, solvents, and other valuable chemicals. The ability to control the reaction conditions, such as the ratio of reactants and temperature, allows for tailoring the product distribution to meet specific industrial needs. []
Disclaimer and Information on In-Vitro Research Products
Please be aware that all articles and product information presented on BenchChem are intended solely for informational purposes. The products available for purchase on BenchChem are specifically designed for in-vitro studies, which are conducted outside of living organisms. In-vitro studies, derived from the Latin term "in glass," involve experiments performed in controlled laboratory settings using cells or tissues. It is important to note that these products are not categorized as medicines or drugs, and they have not received approval from the FDA for the prevention, treatment, or cure of any medical condition, ailment, or disease. We must emphasize that any form of bodily introduction of these products into humans or animals is strictly prohibited by law. It is essential to adhere to these guidelines to ensure compliance with legal and ethical standards in research and experimentation.