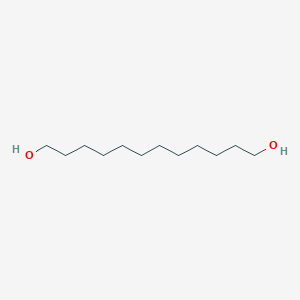
1,12-Dodecanediol
Overview
Description
It is widely used in scientific research to study the function of dopamine receptors in various biological systems . This compound has significant implications in neuroscience, pharmacology, and behavioral studies.
Mechanism of Action
Target of Action
1,12-Dodecanediol is a medium-chain primary fatty alcohol
Biochemical Pathways
This compound has been reported to be a metabolite of Escherichia coli . This suggests that it may be involved in the metabolic pathways of this bacterium.
Pharmacokinetics
Information on the ADME (Absorption, Distribution, Metabolism, and Excretion) properties of this compound is limited. It’s known that the compound has a molecular weight of 202.33 , and it forms crystals at room temperature . Its boiling point is 324 °C/1013 hPa , and it has a melting point of 79 °C . These properties can influence its bioavailability and pharmacokinetics.
Action Environment
The action of this compound can be influenced by various environmental factors. For instance, its stability and efficacy may be affected by temperature, as it forms crystals at room temperature . Additionally, its autoignition temperature is 300 °C , indicating that it can combust at high temperatures. Therefore, the storage and handling conditions can significantly impact the action of this compound.
Biochemical Analysis
Biochemical Properties
1,12-Dodecanediol interacts with various biomolecules in biochemical reactions. For instance, it has been used to synthesize poly(1,12-dodecylene dicarboxylate)s with 1,4-butanedioic acid, dimethyl adipate, dimethyl octanedioate, and 1,10-decanedioic acid . The nature of these interactions involves the formation of ester bonds between the hydroxyl groups of this compound and the carboxyl groups of the dicarboxylic acids .
Cellular Effects
The effects of this compound on cells have been studied in the context of whole-cell biotransformation in Escherichia coli . It was found that the number of living cells dramatically decreased upon the addition of this compound due to its toxicity . By adjusting the temperature and substrate addition, cell growth was restored and this compound production was enhanced .
Molecular Mechanism
The molecular mechanism of this compound involves its conversion into α,ω-dodecanedioic acid via α,ω-dodecanediol in a mutant of Candida tropicalis . This process involves the oxyfunctionalization of n-dodecane by the proteins resulting from the expression of the CYP153A M.aq operon .
Temporal Effects in Laboratory Settings
Over time, the effects of this compound in laboratory settings can change. For instance, in a study involving Escherichia coli, it was observed that the production of this compound increased over a period of 68 hours . This suggests that this compound has a certain level of stability and does not degrade rapidly in in vitro settings .
Metabolic Pathways
This compound is involved in the metabolic pathway that converts n-dodecane to α,ω-dodecanedioic acid . This pathway involves the oxyfunctionalization of n-dodecane, leading to the formation of this compound, which is then further converted into α,ω-dodecanedioic acid .
Preparation Methods
Synthetic Routes and Reaction Conditions
The synthesis of LY-171555 involves several steps. The process begins with the cyclization of 4-benzoyloxycyclohexanone with ethyl 2-bromomethylacrylate and propylamine in refluxing toluene, yielding ethyl 1-propyl-6-benzoyloxy-1,2,3,4,5,6,7,8-octahydroquinoline-3-carboxylate. This intermediate is then reduced with sodium cyanoborohydride in methanol to produce the corresponding decahydro derivative. Subsequent debenzoylation with sodium hydroxide in methanol affords the hydroxyquinoline, which is oxidized with chromium trioxide-pyridine to give ethyl 1-propyl-6-oxodecahydroquinoline-3-carboxylate. The final steps involve cyclization with hydrazine in ethanol and reduction with lithium aluminum hydride in tetrahydrofuran .
Industrial Production Methods
Industrial production methods for LY-171555 are not extensively documented in the public domain. the synthesis typically involves large-scale chemical reactions under controlled conditions to ensure high yield and purity. The process may include the use of automated reactors and purification systems to streamline production and maintain consistency.
Chemical Reactions Analysis
Types of Reactions
LY-171555 undergoes various chemical reactions, including:
Oxidation: The hydroxyquinoline intermediate is oxidized using chromium trioxide-pyridine.
Reduction: Sodium cyanoborohydride and lithium aluminum hydride are used in reduction steps.
Cyclization: Cyclization reactions are performed using hydrazine in ethanol.
Common Reagents and Conditions
Oxidation: Chromium trioxide-pyridine
Reduction: Sodium cyanoborohydride in methanol, lithium aluminum hydride in tetrahydrofuran
Cyclization: Hydrazine in ethanol
Major Products Formed
The major products formed during the synthesis of LY-171555 include various intermediates such as ethyl 1-propyl-6-benzoyloxy-1,2,3,4,5,6,7,8-octahydroquinoline-3-carboxylate and ethyl 1-propyl-6-oxodecahydroquinoline-3-carboxylate, leading to the final product, Quinpirole hydrochloride .
Scientific Research Applications
LY-171555 is extensively used in scientific research due to its high affinity for dopamine D2 and D3 receptors. Some of its applications include:
Neuroscience: Studying the role of dopamine receptors in brain function and behavior.
Pharmacology: Investigating the effects of dopamine receptor agonists and antagonists.
Behavioral Studies: Examining the impact of dopamine receptor activation on animal behavior.
Medical Research: Exploring potential therapeutic applications for conditions such as Parkinson’s disease and schizophrenia.
Comparison with Similar Compounds
LY-171555 is unique due to its high selectivity and affinity for dopamine D2 and D3 receptors. Similar compounds include:
Apomorphine: A non-selective dopamine receptor agonist.
Bromocriptine: A dopamine receptor agonist used in treating Parkinson’s disease.
Lisuride: A dopamine receptor agonist with applications in treating migraine and Parkinson’s disease.
These compounds share some pharmacological properties with LY-171555 but differ in their receptor selectivity and clinical applications.
Properties
IUPAC Name |
dodecane-1,12-diol | |
---|---|---|
Source | PubChem | |
URL | https://pubchem.ncbi.nlm.nih.gov | |
Description | Data deposited in or computed by PubChem | |
InChI |
InChI=1S/C12H26O2/c13-11-9-7-5-3-1-2-4-6-8-10-12-14/h13-14H,1-12H2 | |
Source | PubChem | |
URL | https://pubchem.ncbi.nlm.nih.gov | |
Description | Data deposited in or computed by PubChem | |
InChI Key |
GHLKSLMMWAKNBM-UHFFFAOYSA-N | |
Source | PubChem | |
URL | https://pubchem.ncbi.nlm.nih.gov | |
Description | Data deposited in or computed by PubChem | |
Canonical SMILES |
C(CCCCCCO)CCCCCO | |
Source | PubChem | |
URL | https://pubchem.ncbi.nlm.nih.gov | |
Description | Data deposited in or computed by PubChem | |
Molecular Formula |
C12H26O2 | |
Source | PubChem | |
URL | https://pubchem.ncbi.nlm.nih.gov | |
Description | Data deposited in or computed by PubChem | |
DSSTOX Substance ID |
DTXSID1074919 | |
Record name | 1,12-Dodecanediol | |
Source | EPA DSSTox | |
URL | https://comptox.epa.gov/dashboard/DTXSID1074919 | |
Description | DSSTox provides a high quality public chemistry resource for supporting improved predictive toxicology. | |
Molecular Weight |
202.33 g/mol | |
Source | PubChem | |
URL | https://pubchem.ncbi.nlm.nih.gov | |
Description | Data deposited in or computed by PubChem | |
Physical Description |
Dry Powder, Colorless crystalline solid; [Aldrich MSDS] | |
Record name | 1,12-Dodecanediol | |
Source | EPA Chemicals under the TSCA | |
URL | https://www.epa.gov/chemicals-under-tsca | |
Description | EPA Chemicals under the Toxic Substances Control Act (TSCA) collection contains information on chemicals and their regulations under TSCA, including non-confidential content from the TSCA Chemical Substance Inventory and Chemical Data Reporting. | |
Record name | 1,12-Dodecanediol | |
Source | Haz-Map, Information on Hazardous Chemicals and Occupational Diseases | |
URL | https://haz-map.com/Agents/9823 | |
Description | Haz-Map® is an occupational health database designed for health and safety professionals and for consumers seeking information about the adverse effects of workplace exposures to chemical and biological agents. | |
Explanation | Copyright (c) 2022 Haz-Map(R). All rights reserved. Unless otherwise indicated, all materials from Haz-Map are copyrighted by Haz-Map(R). No part of these materials, either text or image may be used for any purpose other than for personal use. Therefore, reproduction, modification, storage in a retrieval system or retransmission, in any form or by any means, electronic, mechanical or otherwise, for reasons other than personal use, is strictly prohibited without prior written permission. | |
CAS No. |
5675-51-4 | |
Record name | 1,12-Dodecanediol | |
Source | CAS Common Chemistry | |
URL | https://commonchemistry.cas.org/detail?cas_rn=5675-51-4 | |
Description | CAS Common Chemistry is an open community resource for accessing chemical information. Nearly 500,000 chemical substances from CAS REGISTRY cover areas of community interest, including common and frequently regulated chemicals, and those relevant to high school and undergraduate chemistry classes. This chemical information, curated by our expert scientists, is provided in alignment with our mission as a division of the American Chemical Society. | |
Explanation | The data from CAS Common Chemistry is provided under a CC-BY-NC 4.0 license, unless otherwise stated. | |
Record name | 1,12-Dodecanediol | |
Source | ChemIDplus | |
URL | https://pubchem.ncbi.nlm.nih.gov/substance/?source=chemidplus&sourceid=0005675514 | |
Description | ChemIDplus is a free, web search system that provides access to the structure and nomenclature authority files used for the identification of chemical substances cited in National Library of Medicine (NLM) databases, including the TOXNET system. | |
Record name | 1,12-Dodecanediol | |
Source | DTP/NCI | |
URL | https://dtp.cancer.gov/dtpstandard/servlet/dwindex?searchtype=NSC&outputformat=html&searchlist=81250 | |
Description | The NCI Development Therapeutics Program (DTP) provides services and resources to the academic and private-sector research communities worldwide to facilitate the discovery and development of new cancer therapeutic agents. | |
Explanation | Unless otherwise indicated, all text within NCI products is free of copyright and may be reused without our permission. Credit the National Cancer Institute as the source. | |
Record name | 1,12-Dodecanediol | |
Source | EPA Chemicals under the TSCA | |
URL | https://www.epa.gov/chemicals-under-tsca | |
Description | EPA Chemicals under the Toxic Substances Control Act (TSCA) collection contains information on chemicals and their regulations under TSCA, including non-confidential content from the TSCA Chemical Substance Inventory and Chemical Data Reporting. | |
Record name | 1,12-Dodecanediol | |
Source | EPA DSSTox | |
URL | https://comptox.epa.gov/dashboard/DTXSID1074919 | |
Description | DSSTox provides a high quality public chemistry resource for supporting improved predictive toxicology. | |
Record name | Dodecane-1,12-diol | |
Source | European Chemicals Agency (ECHA) | |
URL | https://echa.europa.eu/substance-information/-/substanceinfo/100.024.667 | |
Description | The European Chemicals Agency (ECHA) is an agency of the European Union which is the driving force among regulatory authorities in implementing the EU's groundbreaking chemicals legislation for the benefit of human health and the environment as well as for innovation and competitiveness. | |
Explanation | Use of the information, documents and data from the ECHA website is subject to the terms and conditions of this Legal Notice, and subject to other binding limitations provided for under applicable law, the information, documents and data made available on the ECHA website may be reproduced, distributed and/or used, totally or in part, for non-commercial purposes provided that ECHA is acknowledged as the source: "Source: European Chemicals Agency, http://echa.europa.eu/". Such acknowledgement must be included in each copy of the material. ECHA permits and encourages organisations and individuals to create links to the ECHA website under the following cumulative conditions: Links can only be made to webpages that provide a link to the Legal Notice page. | |
Record name | 1,12-Dodecanediol | |
Source | FDA Global Substance Registration System (GSRS) | |
URL | https://gsrs.ncats.nih.gov/ginas/app/beta/substances/S694C87MF3 | |
Description | The FDA Global Substance Registration System (GSRS) enables the efficient and accurate exchange of information on what substances are in regulated products. Instead of relying on names, which vary across regulatory domains, countries, and regions, the GSRS knowledge base makes it possible for substances to be defined by standardized, scientific descriptions. | |
Explanation | Unless otherwise noted, the contents of the FDA website (www.fda.gov), both text and graphics, are not copyrighted. They are in the public domain and may be republished, reprinted and otherwise used freely by anyone without the need to obtain permission from FDA. Credit to the U.S. Food and Drug Administration as the source is appreciated but not required. | |
Synthesis routes and methods
Procedure details
Retrosynthesis Analysis
AI-Powered Synthesis Planning: Our tool employs the Template_relevance Pistachio, Template_relevance Bkms_metabolic, Template_relevance Pistachio_ringbreaker, Template_relevance Reaxys, Template_relevance Reaxys_biocatalysis model, leveraging a vast database of chemical reactions to predict feasible synthetic routes.
One-Step Synthesis Focus: Specifically designed for one-step synthesis, it provides concise and direct routes for your target compounds, streamlining the synthesis process.
Accurate Predictions: Utilizing the extensive PISTACHIO, BKMS_METABOLIC, PISTACHIO_RINGBREAKER, REAXYS, REAXYS_BIOCATALYSIS database, our tool offers high-accuracy predictions, reflecting the latest in chemical research and data.
Strategy Settings
Precursor scoring | Relevance Heuristic |
---|---|
Min. plausibility | 0.01 |
Model | Template_relevance |
Template Set | Pistachio/Bkms_metabolic/Pistachio_ringbreaker/Reaxys/Reaxys_biocatalysis |
Top-N result to add to graph | 6 |
Feasible Synthetic Routes
Q1: What is the molecular formula and weight of 1,12-dodecanediol?
A1: The molecular formula of this compound is C12H26O2, and its molecular weight is 202.34 g/mol [].
Q2: What spectroscopic techniques are used to characterize this compound?
A2: Researchers commonly use Fourier transform infrared (FTIR) spectroscopy, 1H NMR spectroscopy, and 13C NMR spectroscopy to characterize the chemical structure of this compound and its derivatives [, , , ]. Additionally, GC-MS is used to elucidate the structure of low-molecular weight reaction intermediates during esterification reactions with this compound [].
Q3: What are the key applications of this compound in polymer synthesis?
A3: this compound is frequently used as a monomer or extender in the synthesis of polyesters, polyurethanes, and poly(ester amide)s [, , , , , , , , , , ]. These polymers find applications in various fields, including biomaterials, coatings, and packaging.
Q4: How does the inclusion of this compound as an extender affect the properties of copolyesteraramides?
A4: this compound acts as an extender by increasing the lamellar thickness of bisesterdiamide crystals in copolyesteraramides []. This leads to a multiphase structure with two glass transition temperatures and a broad melting transition, ultimately improving elasticity [].
Q5: How does the chain length of the diol component in polyurethanes, including this compound, affect their properties?
A5: The length of the α,ω-aliphatic glycol extender, such as this compound, significantly impacts the properties of polyurethanes []. Properties like compression set, modulus, and glass transition temperature are notably affected. Optimal properties were observed with this compound and other specific diol chain lengths [].
Q6: What is the role of this compound in the synthesis of biodegradable polymers?
A6: this compound serves as a monomer alongside citric acid and other biocompatible components in the synthesis of biodegradable polyesters [, , , ]. These polyesters exhibit desirable mechanical and degradation properties for potential applications in tissue engineering and drug delivery [, ].
Q7: Does this compound exhibit catalytic properties itself?
A7: this compound primarily serves as a building block or reactant in the reported research. Its direct catalytic properties haven't been a focus in these studies.
Q8: Have there been any molecular dynamics simulations involving this compound?
A8: Yes, molecular dynamics simulations have been used to study the behavior of this compound at the benzene-graphite interface []. These simulations revealed the impact of the adsorbed this compound monolayer on the liquid structure near the interface [].
Q9: How does the structure of this compound relate to its function as a monomer in polymer synthesis?
A9: The linear, long-chain structure of this compound, with terminal hydroxyl groups, makes it suitable for condensation polymerization reactions [, , , ]. The length of the carbon chain influences the flexibility and crystallinity of the resulting polymers, impacting their thermal and mechanical properties [, , , ].
Q10: How does the presence of fluorine atoms in fluorodiol-containing polyurethanes, synthesized using this compound, impact their properties?
A10: Incorporating fluorodiols like 1H,1H,12H,12H-perfluoro-1,12-dodecanediol, derived from this compound, in polyurethanes influences their surface properties and blood compatibility []. Higher fluorine content correlates with lower surface energy, reduced platelet adhesion, and a lower fibrinogen/albumin adsorption ratio, indicating improved blood compatibility [].
Q11: What research has been done on the biocompatibility of this compound-based polymers?
A11: In vitro biocompatibility studies have been conducted on citric acid-based polyesters synthesized using this compound [, ]. These studies demonstrated favorable cell compatibility, suggesting potential applications in tissue engineering and other biomedical fields [, ].
Q12: How does the structure of FDCA-based polyesters, incorporating various polyols like this compound, influence their enzymatic hydrolysis?
A12: The choice of polyol, including this compound, significantly impacts the enzymatic hydrolysis of 2,5-furandicarboxylic acid (FDCA)-based polyesters []. Factors like diol chain length, branching, and the presence of ethoxy units affect the susceptibility of these polyesters to enzymatic degradation by cutinase 1 from Thermobifida cellulosilytica [].
Q13: Are there any studies on the biodegradation of this compound-containing polymers?
A13: While specific biodegradation studies focusing solely on this compound-containing polymers might be limited in the provided research, in vitro degradation studies on citric acid-based polyesters incorporating this compound have been conducted []. These studies provide insights into the degradation behavior of such polymers in a controlled environment [].
Q14: How is this compound typically quantified in various matrices?
A14: Gas chromatography (GC) and related techniques like GC-MS are common methods for quantifying this compound, particularly in the context of analyzing reaction products and intermediates [, , ].
Q15: What is the significance of synthesizing this compound-based bolaamphiphiles?
A15: Bolaamphiphiles, synthesized from this compound and other components, have garnered interest for their ability to form monolayer lipid membranes (MLMs) []. These MLMs exhibit distinct properties compared to traditional bilayer membranes, offering potential in areas like drug delivery, sensing, and biomimetic systems [].
Q16: How is this compound used in the synthesis of the sex pheromone of specific insects?
A16: this compound serves as a starting material in the synthesis of (Z/E)-12-tetradecene-1-ol acetate, a sex pheromone component of insects like the Asian corn borer [, ]. This synthesis involves a series of reactions, including mono-bromination, acylation, oxidation, and a Wittig reaction, showcasing the versatility of this compound as a chemical precursor [, ].
Q17: What are the advantages of using this compound in the synthesis of these pheromones?
A17: this compound offers a cost-effective and readily available starting material for synthesizing these insect pheromones [, ]. The synthetic routes are generally straightforward and efficient, making it a practical choice for pheromone production [, ].
Q18: Can this compound be used to modify the surface of nanomaterials?
A18: Yes, research indicates that this compound can modify the surface of silicon nanocrystallites []. This surface modification can influence the photoluminescent properties of these nanomaterials and potentially open avenues for tailoring their characteristics for specific applications [].
Q19: How does the incorporation of this compound impact the properties of polypropylene/polybutene-1 (PP/PB-1) blends?
A19: When this compound is used to functionalize reduced graphene oxide (rGO) in PP/PB-1 blends, it contributes to improved adhesion and dispersion of both the rGO nanoparticles and the polybutene-1 phase within the polypropylene matrix []. This leads to enhanced mechanical properties, including increased Young's modulus and tensile strength, in the resulting nanocomposites [].
Q20: Are there alternative compounds to this compound for specific applications?
A20: Yes, depending on the specific application, other diols with varying chain lengths or functionalities may be considered as alternatives to this compound [, , , ]. For instance, in polyurethane synthesis, 1,3-propanediol and 1,10-decanediol have been explored as alternative extenders, each imparting different properties to the final polymer [].
Disclaimer and Information on In-Vitro Research Products
Please be aware that all articles and product information presented on BenchChem are intended solely for informational purposes. The products available for purchase on BenchChem are specifically designed for in-vitro studies, which are conducted outside of living organisms. In-vitro studies, derived from the Latin term "in glass," involve experiments performed in controlled laboratory settings using cells or tissues. It is important to note that these products are not categorized as medicines or drugs, and they have not received approval from the FDA for the prevention, treatment, or cure of any medical condition, ailment, or disease. We must emphasize that any form of bodily introduction of these products into humans or animals is strictly prohibited by law. It is essential to adhere to these guidelines to ensure compliance with legal and ethical standards in research and experimentation.