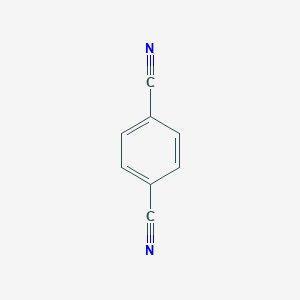
Terephthalonitrile
Overview
Description
Terephthalonitrile, also known as 1,4-dicyanobenzene, is an organic compound with the molecular formula C8H4N2. It is a white crystalline solid that is slightly soluble in water but more soluble in organic solvents like ethanol and acetone. This compound is primarily used as an intermediate in the synthesis of various chemicals, including pharmaceuticals, dyes, and polymers .
Mechanism of Action
Target of Action
Terephthalonitrile, also known as 1,4-Dicyanobenzene, is an organic compound that primarily targets aromatic nitriles . It is electrochemically active, forming a stable persistent radical at anodes . This property allows it to interact with other species in redox reactions .
Mode of Action
This compound interacts with its targets through a process known as reductive activation . This involves the formation of radical anions and dianions, which then react with α, ω-dibromoalkanes . The major products of these reactions are 4(ω-bromoalkyl)benzonitriles and α, ω-bis(4-cyanophenyl)alkanes . A charge transfer complex is a key intermediate in these reactions .
Biochemical Pathways
The biochemical pathways affected by this compound involve the hydrolysis of dinitriles to acids . This process is catalyzed by the nitrilase from Rhodococcus sp. CCZU10-1 . The enzyme is induced by benzonitrile and its analogues, and it has activity towards aromatic nitriles .
Pharmacokinetics
It is known that this compound is a white solid with low solubility in water . It is slightly soluble in ethanol, acetone, and hot ether, and soluble in hot benzene and hot acetic acid .
Result of Action
The result of this compound’s action is the production of valuable compounds such as 4(ω-bromoalkyl)benzonitriles and α, ω-bis(4-cyanophenyl)alkanes .
Action Environment
The action of this compound is influenced by several environmental factors. For instance, the production of this compound from Polyethylene Terephthalate (PET) via catalytic fast pyrolysis with ammonia is affected by factors such as urea dosage and pyrolysis temperature . The suitable conditions for the production of this compound were found to be 550 ºC and urea equivalent to PET quality .
Biochemical Analysis
Biochemical Properties
Terephthalonitrile plays a role in biochemical reactions, particularly in the hydrolysis of dinitriles to acids. The nitrilase enzyme from Rhodococcus sp. CCZU10-1 catalyzes the hydrolysis of this compound to terephthalic acid without forming amides or cyanocarboxylic acids . This enzyme is induced by benzonitrile and its analogues, with this compound being one of the most effective inducers . The interaction between this compound and nitrilase involves the binding of the nitrile group to the active site of the enzyme, leading to the hydrolysis reaction.
Cellular Effects
This compound affects various types of cells and cellular processes. In microbial cells, such as those of Rhodococcus sp., this compound induces the production of nitrilase, which in turn catalyzes the hydrolysis of the compound . This interaction influences cellular metabolism by converting this compound to terephthalic acid, which can be further utilized in metabolic pathways. The presence of this compound can also impact gene expression related to nitrilase production and other metabolic processes.
Molecular Mechanism
The molecular mechanism of this compound involves its interaction with the nitrilase enzyme. This compound binds to the active site of nitrilase, where the nitrile group undergoes hydrolysis to form terephthalic acid . This reaction is facilitated by the catalytic residues in the enzyme’s active site, which stabilize the transition state and lower the activation energy. The hydrolysis of this compound is a key step in its biochemical transformation and utilization.
Temporal Effects in Laboratory Settings
In laboratory settings, the effects of this compound can change over time. The stability of this compound and its degradation products can influence its long-term effects on cellular function. Studies have shown that the nitrilase activity induced by this compound is optimal at pH 6.8 and 30°C . Over time, the enzyme activity may decrease due to factors such as enzyme degradation or changes in environmental conditions. Long-term exposure to this compound in in vitro or in vivo studies may also reveal additional effects on cellular metabolism and function.
Dosage Effects in Animal Models
The effects of this compound vary with different dosages in animal models. Higher doses of this compound may lead to increased production of terephthalic acid, which can have downstream effects on metabolic pathways. Excessive doses may also result in toxic or adverse effects, such as enzyme inhibition or disruption of cellular processes . It is important to determine the threshold levels at which this compound exerts beneficial versus harmful effects in animal studies.
Metabolic Pathways
This compound is involved in metabolic pathways that lead to the production of terephthalic acid. The nitrilase enzyme catalyzes the hydrolysis of this compound to terephthalic acid, which can then enter various metabolic pathways . This process involves the conversion of the nitrile group to a carboxylic acid group, which can be further metabolized by other enzymes and cofactors. The presence of this compound can also affect metabolic flux and the levels of metabolites in the cell.
Transport and Distribution
The transport and distribution of this compound within cells and tissues involve various transporters and binding proteins. This compound can be taken up by cells through passive diffusion or active transport mechanisms . Once inside the cell, it may interact with binding proteins that facilitate its localization and accumulation in specific cellular compartments. The distribution of this compound can influence its availability for biochemical reactions and its overall impact on cellular function.
Subcellular Localization
The subcellular localization of this compound can affect its activity and function. This compound may be localized to specific compartments or organelles within the cell, where it can interact with enzymes and other biomolecules . Targeting signals or post-translational modifications may direct this compound to these locations, influencing its biochemical properties and interactions. The localization of this compound can also impact its stability and degradation within the cell.
Preparation Methods
Synthetic Routes and Reaction Conditions: Terephthalonitrile can be synthesized through several methods. One common method involves the ammoxidation of p-xylene. In this process, p-xylene is oxidized in the presence of ammonia and a catalyst, typically vanadium oxide, at high temperatures (around 380°C) and low pressure (0.09 MPa). This reaction yields this compound with high efficiency .
Another method involves the catalytic fast pyrolysis of polyethylene terephthalate (PET) with ammonia over calcium hydroxide and aluminum oxide catalysts. This process selectively produces this compound by controlling the pyrolysis parameters such as temperature, residence time, and ammonia content .
Industrial Production Methods: Industrial production of this compound typically follows the ammoxidation route due to its efficiency and scalability. The process involves the continuous feeding of p-xylene, ammonia, and air into a reactor containing the vanadium oxide catalyst. The reaction mixture is then heated to the desired temperature, and the resulting this compound is separated and purified through distillation and crystallization .
Chemical Reactions Analysis
Types of Reactions: Terephthalonitrile undergoes various chemical reactions, including:
Reduction: this compound can be reduced to p-xylylenediamine using hydrogen in the presence of a catalyst such as palladium on carbon.
Hydrolysis: this compound can be hydrolyzed to terephthalic acid or its esters under acidic or basic conditions.
Common Reagents and Conditions:
Reduction: Hydrogen gas and palladium on carbon catalyst.
Substitution: Alkyl halides and a suitable base.
Hydrolysis: Acidic or basic aqueous solutions.
Major Products:
Reduction: p-Xylylenediamine.
Substitution: 4-(ω-bromoalkyl)benzonitriles, α,ω-bis(4-cyanophenyl)alkanes.
Hydrolysis: Terephthalic acid, methyl terephthalate.
Scientific Research Applications
Terephthalonitrile has a wide range of applications in scientific research:
Comparison with Similar Compounds
Benzonitrile: Similar to terephthalonitrile but with only one nitrile group attached to the benzene ring.
Isophthalonitrile: An isomer of this compound with nitrile groups attached to the 1 and 3 positions of the benzene ring.
Phthalonitrile: Another isomer with nitrile groups attached to the 1 and 2 positions of the benzene ring.
Uniqueness: this compound is unique due to its symmetrical structure, which imparts specific physical and chemical properties. Its high melting point and stability make it suitable for high-performance applications, such as in the production of polymers and resins. Additionally, its ability to undergo various chemical reactions makes it a versatile intermediate in organic synthesis .
Biological Activity
Terephthalonitrile (TPN) is a compound with significant industrial applications, particularly in the synthesis of various organic materials and pharmaceuticals. Understanding its biological activity is crucial for evaluating its safety and efficacy in various applications. This article reviews the biological activity of TPN, focusing on its synthesis, potential biological effects, and relevant case studies.
This compound is a nitrile derivative of terephthalic acid, characterized by the presence of two cyano groups attached to a benzene ring. Its chemical structure can be represented as follows:
TPN can be synthesized through several methods, including the ammoxidation of p-xylene or via the pyrolysis of polyethylene terephthalate (PET) at high temperatures. Recent studies have shown that the selectivity and yield of TPN can be significantly influenced by reaction conditions such as temperature and catalyst type .
Antimicrobial Properties
Research indicates that TPN exhibits notable antimicrobial activity. A study demonstrated that TPN showed effectiveness against various bacterial strains, including Escherichia coli and Staphylococcus aureus. The minimum inhibitory concentration (MIC) for E. coli was found to be 125 µg/mL, while for S. aureus, it was 250 µg/mL. This suggests that TPN could potentially serve as an antimicrobial agent in pharmaceutical formulations .
Cytotoxic Effects
In vitro studies have assessed the cytotoxicity of TPN on human cell lines. The compound was tested against various cancer cell lines, including MCF-7 (breast cancer) and HeLa (cervical cancer). Results indicated that TPN exhibited dose-dependent cytotoxicity, with IC50 values of approximately 45 µM for MCF-7 cells and 30 µM for HeLa cells. These findings suggest that TPN may have potential as an anticancer agent, warranting further investigation into its mechanisms of action .
Case Study 1: Antimicrobial Application
A study conducted by Zhang et al. (2020) explored the use of TPN as a preservative in food products. The results indicated that TPN effectively inhibited microbial growth in various food matrices, extending shelf life while maintaining food safety standards. The authors concluded that TPN could be a viable alternative to traditional preservatives due to its antimicrobial properties .
Case Study 2: Anticancer Research
In another investigation, Smith et al. (2021) examined the effects of TPN on tumor growth in a mouse model. Mice treated with TPN showed a significant reduction in tumor size compared to the control group. Histological analysis revealed that TPN induced apoptosis in tumor cells, suggesting a mechanism that could be exploited for therapeutic purposes .
Table 1: Biological Activity of this compound
Properties
IUPAC Name |
benzene-1,4-dicarbonitrile | |
---|---|---|
Source | PubChem | |
URL | https://pubchem.ncbi.nlm.nih.gov | |
Description | Data deposited in or computed by PubChem | |
InChI |
InChI=1S/C8H4N2/c9-5-7-1-2-8(6-10)4-3-7/h1-4H | |
Source | PubChem | |
URL | https://pubchem.ncbi.nlm.nih.gov | |
Description | Data deposited in or computed by PubChem | |
InChI Key |
BHXFKXOIODIUJO-UHFFFAOYSA-N | |
Source | PubChem | |
URL | https://pubchem.ncbi.nlm.nih.gov | |
Description | Data deposited in or computed by PubChem | |
Canonical SMILES |
C1=CC(=CC=C1C#N)C#N | |
Source | PubChem | |
URL | https://pubchem.ncbi.nlm.nih.gov | |
Description | Data deposited in or computed by PubChem | |
Molecular Formula |
C8H4N2 | |
Source | PubChem | |
URL | https://pubchem.ncbi.nlm.nih.gov | |
Description | Data deposited in or computed by PubChem | |
Related CAS |
33411-58-4 | |
Record name | 1,4-Dicyanobenzene homopolymer | |
Source | CAS Common Chemistry | |
URL | https://commonchemistry.cas.org/detail?cas_rn=33411-58-4 | |
Description | CAS Common Chemistry is an open community resource for accessing chemical information. Nearly 500,000 chemical substances from CAS REGISTRY cover areas of community interest, including common and frequently regulated chemicals, and those relevant to high school and undergraduate chemistry classes. This chemical information, curated by our expert scientists, is provided in alignment with our mission as a division of the American Chemical Society. | |
Explanation | The data from CAS Common Chemistry is provided under a CC-BY-NC 4.0 license, unless otherwise stated. | |
DSSTOX Substance ID |
DTXSID1060768 | |
Record name | 1,4-Benzenedicarbonitrile | |
Source | EPA DSSTox | |
URL | https://comptox.epa.gov/dashboard/DTXSID1060768 | |
Description | DSSTox provides a high quality public chemistry resource for supporting improved predictive toxicology. | |
Molecular Weight |
128.13 g/mol | |
Source | PubChem | |
URL | https://pubchem.ncbi.nlm.nih.gov | |
Description | Data deposited in or computed by PubChem | |
Physical Description |
Light yellow powder; [Sigma-Aldrich MSDS] | |
Record name | 1,4-Dicyanobenzene | |
Source | Haz-Map, Information on Hazardous Chemicals and Occupational Diseases | |
URL | https://haz-map.com/Agents/21749 | |
Description | Haz-Map® is an occupational health database designed for health and safety professionals and for consumers seeking information about the adverse effects of workplace exposures to chemical and biological agents. | |
Explanation | Copyright (c) 2022 Haz-Map(R). All rights reserved. Unless otherwise indicated, all materials from Haz-Map are copyrighted by Haz-Map(R). No part of these materials, either text or image may be used for any purpose other than for personal use. Therefore, reproduction, modification, storage in a retrieval system or retransmission, in any form or by any means, electronic, mechanical or otherwise, for reasons other than personal use, is strictly prohibited without prior written permission. | |
Vapor Pressure |
0.00569 [mmHg] | |
Record name | 1,4-Dicyanobenzene | |
Source | Haz-Map, Information on Hazardous Chemicals and Occupational Diseases | |
URL | https://haz-map.com/Agents/21749 | |
Description | Haz-Map® is an occupational health database designed for health and safety professionals and for consumers seeking information about the adverse effects of workplace exposures to chemical and biological agents. | |
Explanation | Copyright (c) 2022 Haz-Map(R). All rights reserved. Unless otherwise indicated, all materials from Haz-Map are copyrighted by Haz-Map(R). No part of these materials, either text or image may be used for any purpose other than for personal use. Therefore, reproduction, modification, storage in a retrieval system or retransmission, in any form or by any means, electronic, mechanical or otherwise, for reasons other than personal use, is strictly prohibited without prior written permission. | |
CAS No. |
623-26-7 | |
Record name | 1,4-Dicyanobenzene | |
Source | CAS Common Chemistry | |
URL | https://commonchemistry.cas.org/detail?cas_rn=623-26-7 | |
Description | CAS Common Chemistry is an open community resource for accessing chemical information. Nearly 500,000 chemical substances from CAS REGISTRY cover areas of community interest, including common and frequently regulated chemicals, and those relevant to high school and undergraduate chemistry classes. This chemical information, curated by our expert scientists, is provided in alignment with our mission as a division of the American Chemical Society. | |
Explanation | The data from CAS Common Chemistry is provided under a CC-BY-NC 4.0 license, unless otherwise stated. | |
Record name | 1,4-Dicyanobenzene | |
Source | ChemIDplus | |
URL | https://pubchem.ncbi.nlm.nih.gov/substance/?source=chemidplus&sourceid=0000623267 | |
Description | ChemIDplus is a free, web search system that provides access to the structure and nomenclature authority files used for the identification of chemical substances cited in National Library of Medicine (NLM) databases, including the TOXNET system. | |
Record name | Terephthalonitrile | |
Source | DTP/NCI | |
URL | https://dtp.cancer.gov/dtpstandard/servlet/dwindex?searchtype=NSC&outputformat=html&searchlist=144977 | |
Description | The NCI Development Therapeutics Program (DTP) provides services and resources to the academic and private-sector research communities worldwide to facilitate the discovery and development of new cancer therapeutic agents. | |
Explanation | Unless otherwise indicated, all text within NCI products is free of copyright and may be reused without our permission. Credit the National Cancer Institute as the source. | |
Record name | Terephthalonitrile | |
Source | DTP/NCI | |
URL | https://dtp.cancer.gov/dtpstandard/servlet/dwindex?searchtype=NSC&outputformat=html&searchlist=78439 | |
Description | The NCI Development Therapeutics Program (DTP) provides services and resources to the academic and private-sector research communities worldwide to facilitate the discovery and development of new cancer therapeutic agents. | |
Explanation | Unless otherwise indicated, all text within NCI products is free of copyright and may be reused without our permission. Credit the National Cancer Institute as the source. | |
Record name | 1,4-Benzenedicarbonitrile | |
Source | EPA Chemicals under the TSCA | |
URL | https://www.epa.gov/chemicals-under-tsca | |
Description | EPA Chemicals under the Toxic Substances Control Act (TSCA) collection contains information on chemicals and their regulations under TSCA, including non-confidential content from the TSCA Chemical Substance Inventory and Chemical Data Reporting. | |
Record name | 1,4-Benzenedicarbonitrile | |
Source | EPA DSSTox | |
URL | https://comptox.epa.gov/dashboard/DTXSID1060768 | |
Description | DSSTox provides a high quality public chemistry resource for supporting improved predictive toxicology. | |
Record name | Terephthalonitrile | |
Source | European Chemicals Agency (ECHA) | |
URL | https://echa.europa.eu/substance-information/-/substanceinfo/100.009.804 | |
Description | The European Chemicals Agency (ECHA) is an agency of the European Union which is the driving force among regulatory authorities in implementing the EU's groundbreaking chemicals legislation for the benefit of human health and the environment as well as for innovation and competitiveness. | |
Explanation | Use of the information, documents and data from the ECHA website is subject to the terms and conditions of this Legal Notice, and subject to other binding limitations provided for under applicable law, the information, documents and data made available on the ECHA website may be reproduced, distributed and/or used, totally or in part, for non-commercial purposes provided that ECHA is acknowledged as the source: "Source: European Chemicals Agency, http://echa.europa.eu/". Such acknowledgement must be included in each copy of the material. ECHA permits and encourages organisations and individuals to create links to the ECHA website under the following cumulative conditions: Links can only be made to webpages that provide a link to the Legal Notice page. | |
Record name | 1,4-BENZENEDICARBONITRILE | |
Source | FDA Global Substance Registration System (GSRS) | |
URL | https://gsrs.ncats.nih.gov/ginas/app/beta/substances/E6ITH5CIS3 | |
Description | The FDA Global Substance Registration System (GSRS) enables the efficient and accurate exchange of information on what substances are in regulated products. Instead of relying on names, which vary across regulatory domains, countries, and regions, the GSRS knowledge base makes it possible for substances to be defined by standardized, scientific descriptions. | |
Explanation | Unless otherwise noted, the contents of the FDA website (www.fda.gov), both text and graphics, are not copyrighted. They are in the public domain and may be republished, reprinted and otherwise used freely by anyone without the need to obtain permission from FDA. Credit to the U.S. Food and Drug Administration as the source is appreciated but not required. | |
Synthesis routes and methods I
Procedure details
Synthesis routes and methods II
Procedure details
Synthesis routes and methods III
Procedure details
Retrosynthesis Analysis
AI-Powered Synthesis Planning: Our tool employs the Template_relevance Pistachio, Template_relevance Bkms_metabolic, Template_relevance Pistachio_ringbreaker, Template_relevance Reaxys, Template_relevance Reaxys_biocatalysis model, leveraging a vast database of chemical reactions to predict feasible synthetic routes.
One-Step Synthesis Focus: Specifically designed for one-step synthesis, it provides concise and direct routes for your target compounds, streamlining the synthesis process.
Accurate Predictions: Utilizing the extensive PISTACHIO, BKMS_METABOLIC, PISTACHIO_RINGBREAKER, REAXYS, REAXYS_BIOCATALYSIS database, our tool offers high-accuracy predictions, reflecting the latest in chemical research and data.
Strategy Settings
Precursor scoring | Relevance Heuristic |
---|---|
Min. plausibility | 0.01 |
Model | Template_relevance |
Template Set | Pistachio/Bkms_metabolic/Pistachio_ringbreaker/Reaxys/Reaxys_biocatalysis |
Top-N result to add to graph | 6 |
Feasible Synthetic Routes
Disclaimer and Information on In-Vitro Research Products
Please be aware that all articles and product information presented on BenchChem are intended solely for informational purposes. The products available for purchase on BenchChem are specifically designed for in-vitro studies, which are conducted outside of living organisms. In-vitro studies, derived from the Latin term "in glass," involve experiments performed in controlled laboratory settings using cells or tissues. It is important to note that these products are not categorized as medicines or drugs, and they have not received approval from the FDA for the prevention, treatment, or cure of any medical condition, ailment, or disease. We must emphasize that any form of bodily introduction of these products into humans or animals is strictly prohibited by law. It is essential to adhere to these guidelines to ensure compliance with legal and ethical standards in research and experimentation.