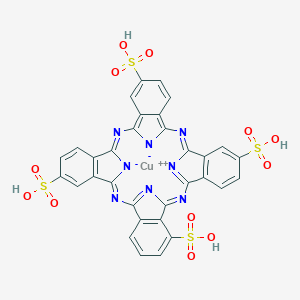
3,4',4'',4'''-Tetrasulfonyl copper phthalocyanine, tetra sodium salt
Overview
Description
3,4’,4’‘,4’‘’-Tetrasulfonyl copper phthalocyanine, tetra sodium salt is a complex organic compound with the molecular formula C32H12CuN8O12S4 · 4Na. It is a derivative of copper phthalocyanine, a well-known pigment, and is characterized by the presence of four sulfonyl groups attached to the phthalocyanine ring. This compound is known for its vibrant color and is used in various applications, including dyes and pigments.
Preparation Methods
Synthetic Routes and Reaction Conditions
The synthesis of 3,4’,4’‘,4’‘’-Tetrasulfonyl copper phthalocyanine, tetra sodium salt typically involves the sulfonation of copper phthalocyanine. The process begins with the preparation of copper phthalocyanine, which is then subjected to sulfonation using sulfuric acid or oleum.
Industrial Production Methods
In industrial settings, the production of this compound follows a similar synthetic route but on a larger scale. The process involves the use of large reactors and precise control of reaction parameters to achieve high yields and purity. The final product is isolated and purified through filtration, washing, and drying processes .
Chemical Reactions Analysis
Types of Reactions
3,4’,4’‘,4’‘’-Tetrasulfonyl copper phthalocyanine, tetra sodium salt undergoes various chemical reactions, including:
Oxidation: The compound can be oxidized under specific conditions to form higher oxidation state derivatives.
Reduction: It can be reduced to lower oxidation state forms using reducing agents.
Substitution: The sulfonyl groups can be substituted with other functional groups through nucleophilic substitution reactions.
Common Reagents and Conditions
Oxidation: Common oxidizing agents include potassium permanganate and hydrogen peroxide.
Reduction: Reducing agents such as sodium borohydride and lithium aluminum hydride are used.
Substitution: Nucleophiles like amines and alcohols can be used for substitution reactions.
Major Products
The major products formed from these reactions depend on the specific reagents and conditions used. For example, oxidation may yield sulfonic acid derivatives, while reduction can produce amine derivatives .
Scientific Research Applications
3,4’,4’‘,4’‘’-Tetrasulfonyl copper phthalocyanine, tetra sodium salt has a wide range of scientific research applications:
Chemistry: Used as a catalyst in various organic reactions due to its stability and reactivity.
Biology: Employed in bioimaging and as a fluorescent marker due to its strong absorption and emission properties.
Medicine: Investigated for its potential use in photodynamic therapy for cancer treatment.
Industry: Widely used as a dye and pigment in textiles, inks, and coatings.
Mechanism of Action
The mechanism of action of 3,4’,4’‘,4’‘’-Tetrasulfonyl copper phthalocyanine, tetra sodium salt involves its interaction with molecular targets through its sulfonyl groups and copper center. The compound can generate reactive oxygen species (ROS) under light irradiation, making it effective in photodynamic therapy. The sulfonyl groups enhance its solubility and facilitate its interaction with biological molecules .
Comparison with Similar Compounds
Similar Compounds
Copper phthalocyanine: The parent compound without sulfonyl groups.
Iron phthalocyanine: Similar structure but with iron as the central metal.
Nickel phthalocyanine: Contains nickel instead of copper.
Uniqueness
3,4’,4’‘,4’‘’-Tetrasulfonyl copper phthalocyanine, tetra sodium salt is unique due to its enhanced solubility and reactivity imparted by the sulfonyl groups. These properties make it more versatile in various applications compared to its non-sulfonated counterparts .
Properties
IUPAC Name |
copper;2,11,20,29,37,40-hexaza-38,39-diazanidanonacyclo[28.6.1.13,10.112,19.121,28.04,9.013,18.022,27.031,36]tetraconta-1(37),2,4(9),5,7,10(40),11,13(18),14,16,19,21,23,25,27,29,31(36),32,34-nonadecaene-5,15,24,33-tetrasulfonic acid | |
---|---|---|
Source | PubChem | |
URL | https://pubchem.ncbi.nlm.nih.gov | |
Description | Data deposited in or computed by PubChem | |
InChI |
InChI=1S/C32H16N8O12S4.Cu/c41-53(42,43)13-4-7-16-20(10-13)29-33-25(16)35-30-22-12-15(55(47,48)49)6-9-18(22)27(37-30)39-32-24-19(2-1-3-23(24)56(50,51)52)28(40-32)38-31-21-11-14(54(44,45)46)5-8-17(21)26(34-29)36-31;/h1-12H,(H4-2,33,34,35,36,37,38,39,40,41,42,43,44,45,46,47,48,49,50,51,52);/q-2;+2 | |
Source | PubChem | |
URL | https://pubchem.ncbi.nlm.nih.gov | |
Description | Data deposited in or computed by PubChem | |
InChI Key |
CMYOSIPFSOPTRC-UHFFFAOYSA-N | |
Source | PubChem | |
URL | https://pubchem.ncbi.nlm.nih.gov | |
Description | Data deposited in or computed by PubChem | |
Canonical SMILES |
C1=CC2=C(C(=C1)S(=O)(=O)O)C3=NC4=NC(=NC5=C6C=CC(=CC6=C([N-]5)N=C7C8=C(C=C(C=C8)S(=O)(=O)O)C(=NC2=N3)[N-]7)S(=O)(=O)O)C9=C4C=CC(=C9)S(=O)(=O)O.[Cu+2] | |
Source | PubChem | |
URL | https://pubchem.ncbi.nlm.nih.gov | |
Description | Data deposited in or computed by PubChem | |
Molecular Formula |
C32H16CuN8O12S4 | |
Source | PubChem | |
URL | https://pubchem.ncbi.nlm.nih.gov | |
Description | Data deposited in or computed by PubChem | |
DSSTOX Substance ID |
DTXSID00154012 | |
Record name | 3,4',4'',4'''-Tetrasulfonyl copper phthalocyanine, tetra sodium salt | |
Source | EPA DSSTox | |
URL | https://comptox.epa.gov/dashboard/DTXSID00154012 | |
Description | DSSTox provides a high quality public chemistry resource for supporting improved predictive toxicology. | |
Molecular Weight |
896.3 g/mol | |
Source | PubChem | |
URL | https://pubchem.ncbi.nlm.nih.gov | |
Description | Data deposited in or computed by PubChem | |
Physical Description |
Dark purple solid; [Sigma-Aldrich MSDS] | |
Record name | Copper phthalocyanine-3,4',4",4"'-tetrasulfonic acid tetrasodium salt | |
Source | Haz-Map, Information on Hazardous Chemicals and Occupational Diseases | |
URL | https://haz-map.com/Agents/20478 | |
Description | Haz-Map® is an occupational health database designed for health and safety professionals and for consumers seeking information about the adverse effects of workplace exposures to chemical and biological agents. | |
Explanation | Copyright (c) 2022 Haz-Map(R). All rights reserved. Unless otherwise indicated, all materials from Haz-Map are copyrighted by Haz-Map(R). No part of these materials, either text or image may be used for any purpose other than for personal use. Therefore, reproduction, modification, storage in a retrieval system or retransmission, in any form or by any means, electronic, mechanical or otherwise, for reasons other than personal use, is strictly prohibited without prior written permission. | |
CAS No. |
123439-80-5 | |
Record name | 3,4',4'',4'''-Tetrasulfonyl copper phthalocyanine, tetra sodium salt | |
Source | ChemIDplus | |
URL | https://pubchem.ncbi.nlm.nih.gov/substance/?source=chemidplus&sourceid=0123439805 | |
Description | ChemIDplus is a free, web search system that provides access to the structure and nomenclature authority files used for the identification of chemical substances cited in National Library of Medicine (NLM) databases, including the TOXNET system. | |
Record name | 3,4',4'',4'''-Tetrasulfonyl copper phthalocyanine, tetra sodium salt | |
Source | EPA DSSTox | |
URL | https://comptox.epa.gov/dashboard/DTXSID00154012 | |
Description | DSSTox provides a high quality public chemistry resource for supporting improved predictive toxicology. | |
Record name | Copper phthalocyanine-3,4',4'',4'''-tetrasulfonic acid tetrasodium salt | |
Source | European Chemicals Agency (ECHA) | |
URL | https://echa.europa.eu/information-on-chemicals | |
Description | The European Chemicals Agency (ECHA) is an agency of the European Union which is the driving force among regulatory authorities in implementing the EU's groundbreaking chemicals legislation for the benefit of human health and the environment as well as for innovation and competitiveness. | |
Explanation | Use of the information, documents and data from the ECHA website is subject to the terms and conditions of this Legal Notice, and subject to other binding limitations provided for under applicable law, the information, documents and data made available on the ECHA website may be reproduced, distributed and/or used, totally or in part, for non-commercial purposes provided that ECHA is acknowledged as the source: "Source: European Chemicals Agency, http://echa.europa.eu/". Such acknowledgement must be included in each copy of the material. ECHA permits and encourages organisations and individuals to create links to the ECHA website under the following cumulative conditions: Links can only be made to webpages that provide a link to the Legal Notice page. | |
Disclaimer and Information on In-Vitro Research Products
Please be aware that all articles and product information presented on BenchChem are intended solely for informational purposes. The products available for purchase on BenchChem are specifically designed for in-vitro studies, which are conducted outside of living organisms. In-vitro studies, derived from the Latin term "in glass," involve experiments performed in controlled laboratory settings using cells or tissues. It is important to note that these products are not categorized as medicines or drugs, and they have not received approval from the FDA for the prevention, treatment, or cure of any medical condition, ailment, or disease. We must emphasize that any form of bodily introduction of these products into humans or animals is strictly prohibited by law. It is essential to adhere to these guidelines to ensure compliance with legal and ethical standards in research and experimentation.