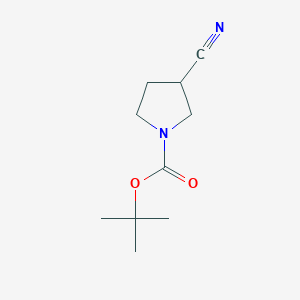
1-N-Boc-3-Cyanopyrrolidine
Overview
Description
Synthesis Analysis
The synthesis of 1-N-Boc-3-Cyanopyrrolidine-related compounds involves lithiation-substitution reactions. Sheikh et al. (2012) detailed the enantioselective synthesis of 2-substituted 2-phenylpyrrolidines and -piperidines, an important class of compounds that contain a quaternary stereocenter. They used lithiation-substitution of N-Boc-2-phenylpyrrolidine or -piperidine prepared by asymmetric Negishi arylation or catalytic asymmetric reduction (Sheikh et al., 2012).
Molecular Structure Analysis
The molecular structure of N-Boc-protected pyrrolidine derivatives and their analogs can be elucidated using spectroscopic techniques like NMR and X-ray crystallography. For example, Matulevičiūtė et al. (2023) synthesized new N-(aminocycloalkylene)amino acid derivatives and elucidated their structure using these techniques (Matulevičiūtė et al., 2023).
Chemical Reactions and Properties
1-N-Boc-3-Cyanopyrrolidine and similar compounds can undergo various chemical reactions, including 1,3-dipolar cycloadditions. López-Pérez et al. (2011) described the Ag-catalyzed 1,3-dipolar cycloaddition of β-borylacrylates with azomethine ylides, leading to 3-borylpyrrolidine derivatives (López-Pérez et al., 2011).
Physical Properties Analysis
The physical properties of N-Boc-3-Cyanopyrrolidine and related compounds, such as melting points, boiling points, and solubility, can be characterized using standard laboratory techniques. However, specific studies focusing on these physical properties were not found in the current literature search.
Chemical Properties Analysis
The chemical properties, including reactivity and stability, of 1-N-Boc-3-Cyanopyrrolidine analogs can be influenced by factors like the presence of functional groups and stereochemistry. Haddad and Larchevêque (2005) demonstrated the kinetic resolution of N-Boc protected pyrrolidine derivatives through acylation, highlighting the influence of stereochemistry on chemical reactivity (Haddad & Larchevêque, 2005).
Scientific Research Applications
Chemoenzymatic Synthesis : N-Boc protected 3-hydroxy-2-hydroxymethyl-3-methylpyrrolidine was synthesized through a kinetic resolution process involving acylation, catalyzed by Pseudomonas fluorescens lipase. This method achieved high diastereo- and enantioselectivity (Haddad & Larchevêque, 2005).
Lithiation-Substitution Studies : Research on the lithiation-substitution of N-Boc-2-phenylpyrrolidine and -piperidine has led to a general and enantioselective synthesis of 2-substituted 2-phenylpyrrolidines and -piperidines, important for pharmaceutical development. The study utilized in situ IR spectroscopy for monitoring and optimizing lithiation conditions (Sheikh et al., 2012).
Organocatalytic Asymmetric Formal [3 + 2] Cycloaddition : A novel cycloaddition reaction with in situ generation of N-carbamoyl nitrones was developed, using N-Boc-protected isoxazolidines. This method provided valuable building blocks for pharmaceuticals and showcased the use of N-carbamoyl nitrones in asymmetric catalysis (Gioia et al., 2009).
ω-Transaminase-Catalyzed Kinetic Resolutions : In this study, the kinetic resolution of 3-aminopyrrolidine and 3-aminopiperidine was enhanced using a protecting group concept. N-Boc protection increased the reaction rate and enantioselectivity significantly, offering insights into efficient enantiomer separation methods (Höhne et al., 2008).
Synthesis of Cyanopyrrolidines as DPP-IV Inhibitors : Cyanopyrrolidines have been explored as inhibitors of the dipeptidyl peptidase IV enzyme, a target for type 2 diabetes treatment. Research over 11 years culminated in the discovery of vildagliptin and saxagliptin, highlighting the significance of cyanopyrrolidines in diabetes research (Peters, 2007).
Conformational Analysis of β-Lactam-Containing Ferrocene Peptides : This study involved the conjugation of 3-amino-1-(4-methoxyphenyl)-4-phenyl-beta-lactam with Boc-Ala, leading to the formation of ferrocene-based peptides. The research provided insights into the stabilization of these compounds in solution through intramolecular hydrogen bonds (Kovač et al., 2009).
Safety And Hazards
properties
IUPAC Name |
tert-butyl 3-cyanopyrrolidine-1-carboxylate | |
---|---|---|
Source | PubChem | |
URL | https://pubchem.ncbi.nlm.nih.gov | |
Description | Data deposited in or computed by PubChem | |
InChI |
InChI=1S/C10H16N2O2/c1-10(2,3)14-9(13)12-5-4-8(6-11)7-12/h8H,4-5,7H2,1-3H3 | |
Source | PubChem | |
URL | https://pubchem.ncbi.nlm.nih.gov | |
Description | Data deposited in or computed by PubChem | |
InChI Key |
VDDMCMFPUSCJNA-UHFFFAOYSA-N | |
Source | PubChem | |
URL | https://pubchem.ncbi.nlm.nih.gov | |
Description | Data deposited in or computed by PubChem | |
Canonical SMILES |
CC(C)(C)OC(=O)N1CCC(C1)C#N | |
Source | PubChem | |
URL | https://pubchem.ncbi.nlm.nih.gov | |
Description | Data deposited in or computed by PubChem | |
Molecular Formula |
C10H16N2O2 | |
Source | PubChem | |
URL | https://pubchem.ncbi.nlm.nih.gov | |
Description | Data deposited in or computed by PubChem | |
DSSTOX Substance ID |
DTXSID30373537 | |
Record name | 1-N-Boc-3-Cyanopyrrolidine | |
Source | EPA DSSTox | |
URL | https://comptox.epa.gov/dashboard/DTXSID30373537 | |
Description | DSSTox provides a high quality public chemistry resource for supporting improved predictive toxicology. | |
Molecular Weight |
196.25 g/mol | |
Source | PubChem | |
URL | https://pubchem.ncbi.nlm.nih.gov | |
Description | Data deposited in or computed by PubChem | |
Product Name |
1-N-Boc-3-Cyanopyrrolidine | |
CAS RN |
476493-40-0 | |
Record name | 1-N-Boc-3-Cyanopyrrolidine | |
Source | EPA DSSTox | |
URL | https://comptox.epa.gov/dashboard/DTXSID30373537 | |
Description | DSSTox provides a high quality public chemistry resource for supporting improved predictive toxicology. | |
Record name | 1-Boc-3-cyanopyrrolidine | |
Source | European Chemicals Agency (ECHA) | |
URL | https://echa.europa.eu/information-on-chemicals | |
Description | The European Chemicals Agency (ECHA) is an agency of the European Union which is the driving force among regulatory authorities in implementing the EU's groundbreaking chemicals legislation for the benefit of human health and the environment as well as for innovation and competitiveness. | |
Explanation | Use of the information, documents and data from the ECHA website is subject to the terms and conditions of this Legal Notice, and subject to other binding limitations provided for under applicable law, the information, documents and data made available on the ECHA website may be reproduced, distributed and/or used, totally or in part, for non-commercial purposes provided that ECHA is acknowledged as the source: "Source: European Chemicals Agency, http://echa.europa.eu/". Such acknowledgement must be included in each copy of the material. ECHA permits and encourages organisations and individuals to create links to the ECHA website under the following cumulative conditions: Links can only be made to webpages that provide a link to the Legal Notice page. | |
Synthesis routes and methods
Procedure details
Retrosynthesis Analysis
AI-Powered Synthesis Planning: Our tool employs the Template_relevance Pistachio, Template_relevance Bkms_metabolic, Template_relevance Pistachio_ringbreaker, Template_relevance Reaxys, Template_relevance Reaxys_biocatalysis model, leveraging a vast database of chemical reactions to predict feasible synthetic routes.
One-Step Synthesis Focus: Specifically designed for one-step synthesis, it provides concise and direct routes for your target compounds, streamlining the synthesis process.
Accurate Predictions: Utilizing the extensive PISTACHIO, BKMS_METABOLIC, PISTACHIO_RINGBREAKER, REAXYS, REAXYS_BIOCATALYSIS database, our tool offers high-accuracy predictions, reflecting the latest in chemical research and data.
Strategy Settings
Precursor scoring | Relevance Heuristic |
---|---|
Min. plausibility | 0.01 |
Model | Template_relevance |
Template Set | Pistachio/Bkms_metabolic/Pistachio_ringbreaker/Reaxys/Reaxys_biocatalysis |
Top-N result to add to graph | 6 |
Feasible Synthetic Routes
Disclaimer and Information on In-Vitro Research Products
Please be aware that all articles and product information presented on BenchChem are intended solely for informational purposes. The products available for purchase on BenchChem are specifically designed for in-vitro studies, which are conducted outside of living organisms. In-vitro studies, derived from the Latin term "in glass," involve experiments performed in controlled laboratory settings using cells or tissues. It is important to note that these products are not categorized as medicines or drugs, and they have not received approval from the FDA for the prevention, treatment, or cure of any medical condition, ailment, or disease. We must emphasize that any form of bodily introduction of these products into humans or animals is strictly prohibited by law. It is essential to adhere to these guidelines to ensure compliance with legal and ethical standards in research and experimentation.