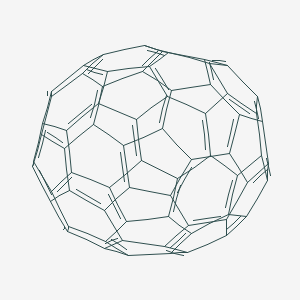
Fullerene C70
Overview
Description
Fullerene C70 is a molecule consisting of 70 carbon atoms. It has a cage-like fused-ring structure that resembles a rugby ball, made of 25 hexagons and 12 pentagons, with a carbon atom at the vertices of each polygon and a bond along each polygon edge . It was first intentionally prepared in 1985 by Harold Kroto, James R. Heath, Sean O’Brien, Robert Curl, and Richard Smalley at Rice University .
Synthesis Analysis
Fullerene C70 synthesis has been achieved through various methods. One method involves the combustion of hydrocarbons in a flat flame burner . Another method involves the use of ethylene and benzene . Monoadducts were obtained with yields of 69% and 44% for C60 and C70 fullerene cages, respectively .
Molecular Structure Analysis
The molecular structure of C70 has been studied using high-Q pulsed powder neutron diffraction of solid C70 . The intra- and inter-molecular pair correlation functions were decoupled, allowing accurate determination of the intramolecular structure of C70 .
Chemical Reactions Analysis
The chemical reactivity of fullerenes is similar to that of a fairly localized, electron-deficient polyolefin . The main reactions are cycloadditions, radical additions, and nucleophilic additions . In the case of monoaddition, C70 has eight possible isomers, including the predominant 1,2- and 5,6-additions .
Physical And Chemical Properties Analysis
Fullerene C70 has a molar mass of 840.770 g·mol −1, appears as dark needle-like crystals, and is insoluble in water . It has a band gap of 1.77 eV . The dipole moment increases almost linearly from 0.005 to 65.005 Debye and the energy gap decreases continuously under the external electric field .
Scientific Research Applications
Organic Photovoltaics
Fullerene C70 is used in organic photovoltaics (OPV) . The unique physical and chemical properties of C70 make it an ideal candidate for use in OPV. The high electron affinity and excellent electron-transport properties of C70 contribute to the high power conversion efficiency of OPV devices .
Water Purification
Fullerene C70 has been used in water purification technologies . The large surface area and the ability to form stable suspensions make C70 an effective adsorbent for removing pollutants from water .
Biohazard Protection
C70 fullerenes have been used in biohazard protection . The antimicrobial properties of C70 make it useful in the development of protective clothing and surfaces .
Medicine
In the field of medicine , C70 has shown potential for various applications . Its unique properties, such as size, hydrophobicity, and electronic configuration, have brought them to the front burner of medical chemistry .
Tribological Materials
C70 is used in the production of tribological materials . These are materials that are used in systems where surfaces move relative to each other, such as in bearings. The unique properties of C70 contribute to the reduction of friction and wear .
Coatings
C70 is used in the production of various coatings . The high thermal stability and resistance to chemical attack make C70 an ideal material for protective coatings .
Electromagnetic Devices
C70 is used as the main building block in electromagnetic devices . Its unique electronic properties make it suitable for use in a variety of devices, including sensors and solar panels .
Portable Power
C70 is used in portable power applications . Its high electron mobility and good thermal stability make it an ideal material for use in batteries and supercapacitors .
Mechanism of Action
Target of Action
Fullerene C70, a molecule consisting of 70 carbon atoms, is a cage-like fused-ring structure . It interacts with various targets due to its unique physicochemical properties. For instance, it has been reported to interact with graphene sheets, forming novel fullerene C70/carbon nanoscroll core/shell composite nanostructures . Moreover, it has been used to form cocrystals with other molecules, such as NiII(OEP), where OEP is the dianion of octaethylporphyrin .
Mode of Action
The mode of action of Fullerene C70 is primarily through its interaction with its targets. For example, in the case of graphene sheets, the fullerene C70 can induce the self-assemble CNS to form a shell–core structure . The interaction between C70 and its targets often involves van der Waals forces and π–π stacking interactions . These interactions are crucial for the formation of various structures and complexes involving C70.
Biochemical Pathways
It has been suggested that fullerenes can participate in reactive oxygen species (ros) homeostasis . This implies that C70 may interact with biochemical pathways related to oxidative stress and inflammation. More research is needed to fully elucidate the biochemical pathways affected by C70.
Pharmacokinetics
It is known that fullerenes are soluble in a variety of organic solvents , which could influence their bioavailability and distribution in the body
Result of Action
The molecular and cellular effects of Fullerene C70’s action are still under investigation. Some studies suggest that C70 can participate in ROS homeostasis, potentially influencing cellular processes related to oxidative stress . Moreover, it has been reported that C70 can induce the formation of novel structures when interacting with other molecules , which could have various cellular effects.
Action Environment
The action, efficacy, and stability of Fullerene C70 can be influenced by various environmental factors. For instance, the interaction between C70 and other molecules can be affected by the solvent used . Moreover, factors such as temperature and pressure can influence the formation of structures involving C70 . Therefore, the environment plays a crucial role in the action of C70.
Safety and Hazards
Future Directions
Fullerene C70 has a broad application prospect. It is of great significance for investigating the properties of fullerene C70 under the external electric field . The absorption peak of fullerene C70 occurs red shift from 504.78 to 736.39 nm . The excitation energy decreases rapidly and the excitation wavelength increases a lot with the external electric field . These achievements will translate into all fields where fullerenes have traditionally and more recently been applied .
properties
IUPAC Name |
(C70-D5h(6))[5,6]fullerene | |
---|---|---|
Source | PubChem | |
URL | https://pubchem.ncbi.nlm.nih.gov | |
Description | Data deposited in or computed by PubChem | |
InChI |
InChI=1S/C70/c1-2-22-5-6-24-13-14-26-11-9-23-4-3(21(1)51-52(22)54(24)55(26)53(23)51)33-31(1)61-35-7-8-27-15-16-29-19-20-30-18-17-28-12-10(25(7)56-57(27)59(29)60(30)58(28)56)37(35)63(33)65-36(4)40(9)67(44(17)42(12)65)69-46(11)47(14)70(50(20)49(18)69)68-43(13)39(6)66(45(16)48(19)68)64-34(5)32(2)62(61)38(8)41(15)64 | |
Source | PubChem | |
URL | https://pubchem.ncbi.nlm.nih.gov | |
Description | Data deposited in or computed by PubChem | |
InChI Key |
ATLMFJTZZPOKLC-UHFFFAOYSA-N | |
Source | PubChem | |
URL | https://pubchem.ncbi.nlm.nih.gov | |
Description | Data deposited in or computed by PubChem | |
Canonical SMILES |
C12=C3C4=C5C6=C7C8=C9C%10=C%11C%12=C%13C%10=C%10C8=C5C1=C%10C1=C%13C5=C8C1=C2C1=C3C2=C3C%10=C%13C%14=C3C1=C8C1=C3C5=C%12C5=C8C%11=C%11C9=C7C7=C9C6=C4C2=C2C%10=C4C(=C29)C2=C6C(=C8C8=C9C6=C4C%13=C9C(=C%141)C3=C85)C%11=C27 | |
Source | PubChem | |
URL | https://pubchem.ncbi.nlm.nih.gov | |
Description | Data deposited in or computed by PubChem | |
Molecular Formula |
C70 | |
Source | PubChem | |
URL | https://pubchem.ncbi.nlm.nih.gov | |
Description | Data deposited in or computed by PubChem | |
Related CAS |
157069-59-5 | |
Record name | [5,6]Fullerene-C70-D5h(6), homopolymer | |
Source | CAS Common Chemistry | |
URL | https://commonchemistry.cas.org/detail?cas_rn=157069-59-5 | |
Description | CAS Common Chemistry is an open community resource for accessing chemical information. Nearly 500,000 chemical substances from CAS REGISTRY cover areas of community interest, including common and frequently regulated chemicals, and those relevant to high school and undergraduate chemistry classes. This chemical information, curated by our expert scientists, is provided in alignment with our mission as a division of the American Chemical Society. | |
Explanation | The data from CAS Common Chemistry is provided under a CC-BY-NC 4.0 license, unless otherwise stated. | |
DSSTOX Substance ID |
DTXSID90151050 | |
Record name | Fullerene C70 | |
Source | EPA DSSTox | |
URL | https://comptox.epa.gov/dashboard/DTXSID90151050 | |
Description | DSSTox provides a high quality public chemistry resource for supporting improved predictive toxicology. | |
Molecular Weight |
840.7 g/mol | |
Source | PubChem | |
URL | https://pubchem.ncbi.nlm.nih.gov | |
Description | Data deposited in or computed by PubChem | |
Product Name |
Fullerene C70 | |
CAS RN |
115383-22-7 | |
Record name | Fullerene-C70 | |
Source | CAS Common Chemistry | |
URL | https://commonchemistry.cas.org/detail?cas_rn=115383-22-7 | |
Description | CAS Common Chemistry is an open community resource for accessing chemical information. Nearly 500,000 chemical substances from CAS REGISTRY cover areas of community interest, including common and frequently regulated chemicals, and those relevant to high school and undergraduate chemistry classes. This chemical information, curated by our expert scientists, is provided in alignment with our mission as a division of the American Chemical Society. | |
Explanation | The data from CAS Common Chemistry is provided under a CC-BY-NC 4.0 license, unless otherwise stated. | |
Record name | Fullerene C70 | |
Source | ChemIDplus | |
URL | https://pubchem.ncbi.nlm.nih.gov/substance/?source=chemidplus&sourceid=0115383227 | |
Description | ChemIDplus is a free, web search system that provides access to the structure and nomenclature authority files used for the identification of chemical substances cited in National Library of Medicine (NLM) databases, including the TOXNET system. | |
Record name | Fullerene C70 | |
Source | EPA DSSTox | |
URL | https://comptox.epa.gov/dashboard/DTXSID90151050 | |
Description | DSSTox provides a high quality public chemistry resource for supporting improved predictive toxicology. | |
Disclaimer and Information on In-Vitro Research Products
Please be aware that all articles and product information presented on BenchChem are intended solely for informational purposes. The products available for purchase on BenchChem are specifically designed for in-vitro studies, which are conducted outside of living organisms. In-vitro studies, derived from the Latin term "in glass," involve experiments performed in controlled laboratory settings using cells or tissues. It is important to note that these products are not categorized as medicines or drugs, and they have not received approval from the FDA for the prevention, treatment, or cure of any medical condition, ailment, or disease. We must emphasize that any form of bodily introduction of these products into humans or animals is strictly prohibited by law. It is essential to adhere to these guidelines to ensure compliance with legal and ethical standards in research and experimentation.