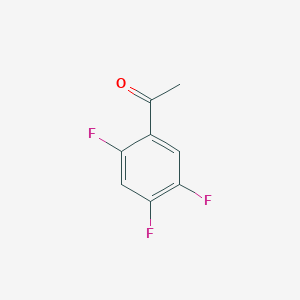
2',4',5'-Trifluoroacetophenone
Overview
Description
2’,4’,5’-Trifluoroacetophenone is an organic compound with the molecular formula C8H5F3O. It is a derivative of acetophenone where three hydrogen atoms on the benzene ring are replaced by fluorine atoms at the 2’, 4’, and 5’ positions. This compound is known for its unique chemical properties and is used in various chemical syntheses and industrial applications.
Mechanism of Action
Target of Action
It is often used as a building block in chemical synthesis , suggesting that it may interact with a variety of molecular targets depending on the specific context of the reaction.
Action Environment
The action, efficacy, and stability of 2’,4’,5’-Trifluoroacetophenone can be influenced by various environmental factors. For instance, the temperature and pH can affect the rate and outcome of the reactions it participates in . Additionally, its stability could be affected by exposure to light, heat, or certain chemicals.
Preparation Methods
Synthetic Routes and Reaction Conditions: 2’,4’,5’-Trifluoroacetophenone can be synthesized through several methods. One common method involves the Friedel-Crafts acylation of 2,4,5-trifluorobenzene with acetyl chloride in the presence of a Lewis acid catalyst such as aluminum chloride. The reaction typically occurs under anhydrous conditions to prevent the hydrolysis of the catalyst.
Industrial Production Methods: In industrial settings, the production of 2’,4’,5’-Trifluoroacetophenone often involves large-scale Friedel-Crafts acylation processes. The reaction conditions are optimized to maximize yield and purity, and the product is purified through distillation or recrystallization techniques.
Chemical Reactions Analysis
Types of Reactions: 2’,4’,5’-Trifluoroacetophenone undergoes various chemical reactions, including:
Oxidation: It can be oxidized to form trifluoroacetic acid derivatives.
Reduction: The carbonyl group can be reduced to form the corresponding alcohol.
Substitution: The fluorine atoms can be substituted with other functional groups under appropriate conditions.
Common Reagents and Conditions:
Oxidation: Common oxidizing agents include potassium permanganate and chromium trioxide.
Reduction: Reducing agents such as sodium borohydride or lithium aluminum hydride are used.
Substitution: Nucleophilic substitution reactions can be carried out using reagents like sodium methoxide or potassium tert-butoxide.
Major Products:
Oxidation: Trifluoroacetic acid derivatives.
Reduction: 2’,4’,5’-Trifluoro-1-phenylethanol.
Substitution: Various substituted acetophenones depending on the nucleophile used.
Scientific Research Applications
2’,4’,5’-Trifluoroacetophenone has several applications in scientific research:
Chemistry: It is used as a building block in the synthesis of more complex organic molecules, including pharmaceuticals and agrochemicals.
Biology: It serves as a precursor in the synthesis of biologically active compounds.
Medicine: The compound is investigated for its potential use in drug development, particularly in the design of enzyme inhibitors.
Industry: It is used in the production of specialty chemicals and materials with unique properties.
Comparison with Similar Compounds
2,2,2-Trifluoroacetophenone: Similar in structure but with all three fluorine atoms on the same carbon.
4’-Chloro-2,2,2-trifluoroacetophenone: Contains a chlorine atom in addition to the trifluoromethyl group.
2’,4’,6’-Trifluoroacetophenone: Fluorine atoms are positioned differently on the benzene ring.
Uniqueness: 2’,4’,5’-Trifluoroacetophenone is unique due to the specific positioning of the fluorine atoms, which imparts distinct electronic and steric properties. This makes it particularly useful in the synthesis of compounds where precise control over reactivity and selectivity is required.
Properties
IUPAC Name |
1-(2,4,5-trifluorophenyl)ethanone | |
---|---|---|
Source | PubChem | |
URL | https://pubchem.ncbi.nlm.nih.gov | |
Description | Data deposited in or computed by PubChem | |
InChI |
InChI=1S/C8H5F3O/c1-4(12)5-2-7(10)8(11)3-6(5)9/h2-3H,1H3 | |
Source | PubChem | |
URL | https://pubchem.ncbi.nlm.nih.gov | |
Description | Data deposited in or computed by PubChem | |
InChI Key |
GVTLJUZWNNFHMZ-UHFFFAOYSA-N | |
Source | PubChem | |
URL | https://pubchem.ncbi.nlm.nih.gov | |
Description | Data deposited in or computed by PubChem | |
Canonical SMILES |
CC(=O)C1=CC(=C(C=C1F)F)F | |
Source | PubChem | |
URL | https://pubchem.ncbi.nlm.nih.gov | |
Description | Data deposited in or computed by PubChem | |
Molecular Formula |
C8H5F3O | |
Source | PubChem | |
URL | https://pubchem.ncbi.nlm.nih.gov | |
Description | Data deposited in or computed by PubChem | |
DSSTOX Substance ID |
DTXSID20344099 | |
Record name | 2',4',5'-Trifluoroacetophenone | |
Source | EPA DSSTox | |
URL | https://comptox.epa.gov/dashboard/DTXSID20344099 | |
Description | DSSTox provides a high quality public chemistry resource for supporting improved predictive toxicology. | |
Molecular Weight |
174.12 g/mol | |
Source | PubChem | |
URL | https://pubchem.ncbi.nlm.nih.gov | |
Description | Data deposited in or computed by PubChem | |
CAS No. |
129322-83-4 | |
Record name | 1-(2,4,5-Trifluorophenyl)ethanone | |
Source | CAS Common Chemistry | |
URL | https://commonchemistry.cas.org/detail?cas_rn=129322-83-4 | |
Description | CAS Common Chemistry is an open community resource for accessing chemical information. Nearly 500,000 chemical substances from CAS REGISTRY cover areas of community interest, including common and frequently regulated chemicals, and those relevant to high school and undergraduate chemistry classes. This chemical information, curated by our expert scientists, is provided in alignment with our mission as a division of the American Chemical Society. | |
Explanation | The data from CAS Common Chemistry is provided under a CC-BY-NC 4.0 license, unless otherwise stated. | |
Record name | 2',4',5'-Trifluoroacetophenone | |
Source | EPA DSSTox | |
URL | https://comptox.epa.gov/dashboard/DTXSID20344099 | |
Description | DSSTox provides a high quality public chemistry resource for supporting improved predictive toxicology. | |
Record name | 2',4',5'-Trifluoroacetophenone | |
Source | European Chemicals Agency (ECHA) | |
URL | https://echa.europa.eu/information-on-chemicals | |
Description | The European Chemicals Agency (ECHA) is an agency of the European Union which is the driving force among regulatory authorities in implementing the EU's groundbreaking chemicals legislation for the benefit of human health and the environment as well as for innovation and competitiveness. | |
Explanation | Use of the information, documents and data from the ECHA website is subject to the terms and conditions of this Legal Notice, and subject to other binding limitations provided for under applicable law, the information, documents and data made available on the ECHA website may be reproduced, distributed and/or used, totally or in part, for non-commercial purposes provided that ECHA is acknowledged as the source: "Source: European Chemicals Agency, http://echa.europa.eu/". Such acknowledgement must be included in each copy of the material. ECHA permits and encourages organisations and individuals to create links to the ECHA website under the following cumulative conditions: Links can only be made to webpages that provide a link to the Legal Notice page. | |
Synthesis routes and methods
Procedure details
Retrosynthesis Analysis
AI-Powered Synthesis Planning: Our tool employs the Template_relevance Pistachio, Template_relevance Bkms_metabolic, Template_relevance Pistachio_ringbreaker, Template_relevance Reaxys, Template_relevance Reaxys_biocatalysis model, leveraging a vast database of chemical reactions to predict feasible synthetic routes.
One-Step Synthesis Focus: Specifically designed for one-step synthesis, it provides concise and direct routes for your target compounds, streamlining the synthesis process.
Accurate Predictions: Utilizing the extensive PISTACHIO, BKMS_METABOLIC, PISTACHIO_RINGBREAKER, REAXYS, REAXYS_BIOCATALYSIS database, our tool offers high-accuracy predictions, reflecting the latest in chemical research and data.
Strategy Settings
Precursor scoring | Relevance Heuristic |
---|---|
Min. plausibility | 0.01 |
Model | Template_relevance |
Template Set | Pistachio/Bkms_metabolic/Pistachio_ringbreaker/Reaxys/Reaxys_biocatalysis |
Top-N result to add to graph | 6 |
Feasible Synthetic Routes
Disclaimer and Information on In-Vitro Research Products
Please be aware that all articles and product information presented on BenchChem are intended solely for informational purposes. The products available for purchase on BenchChem are specifically designed for in-vitro studies, which are conducted outside of living organisms. In-vitro studies, derived from the Latin term "in glass," involve experiments performed in controlled laboratory settings using cells or tissues. It is important to note that these products are not categorized as medicines or drugs, and they have not received approval from the FDA for the prevention, treatment, or cure of any medical condition, ailment, or disease. We must emphasize that any form of bodily introduction of these products into humans or animals is strictly prohibited by law. It is essential to adhere to these guidelines to ensure compliance with legal and ethical standards in research and experimentation.