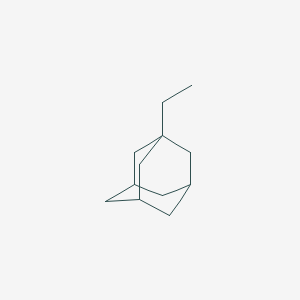
1-Ethyladamantane
Overview
Description
1-Ethyladamantane (CAS Number: 770-69-4) is a chemical compound with the molecular formula C12H20 . It has an average mass of 164.287 Da and a Monoisotopic mass of 164.156494 Da .
Molecular Structure Analysis
The molecular structure of 1-Ethyladamantane consists of a unique arrangement of carbon and hydrogen atoms. It has no hydrogen bond acceptors, no hydrogen bond donors, and one freely rotating bond . The compound’s polarizability is 20.4±0.5 10^-24 cm^3, and it has a molar volume of 171.8±3.0 cm^3 .
Physical And Chemical Properties Analysis
1-Ethyladamantane has a density of 1.0±0.1 g/cm^3, a boiling point of 214.1±7.0 °C at 760 mmHg, and a vapor pressure of 0.2±0.2 mmHg at 25°C . Its enthalpy of vaporization is 43.2±0.8 kJ/mol, and it has a flash point of 69.7±11.7 °C . The compound’s index of refraction is 1.511, and its molar refractivity is 51.5±0.3 cm^3 .
Scientific Research Applications
Quantum-Chemical Studies
1-Ethyladamantane has been used in quantum-chemical studies to understand the formation of alkyl- and alkenyladamantanes by ionic alkylation with olefins . The thermodynamic parameters of formation reactions were estimated, and the effect of methyl groups in adamantanes and the molecular weight of the olefin on the energetics of formation of the corresponding alkyl- and alkenyladamantanes was demonstrated .
Heat Resistance Applications
Adamantanes and alkyladamantanes, including 1-Ethyladamantane, are known for their increased heat resistance . This makes them suitable for applications that require materials to withstand high temperatures.
Stability Applications
1-Ethyladamantane exhibits stability against oxidation, hydrolysis, and exposure to light . This stability makes it useful in various applications where these properties are required.
High Reactivity Applications
The high reactivity of 1-Ethyladamantane makes it a valuable compound in scientific research and industry . It can be used to obtain thermostable and energy-intensive fuels .
Medical Applications
1-Ethyladamantane can be used as a starting compound for the synthesis of drugs . For example, the well-known drug memantine for the treatment of dementia is obtained from 1,3-dimethyladamantane .
Optics and Semiconductor Applications
1-Ethyladamantane can be used in the production of materials for optics . It can also be used in the creation of organic semiconductor luminophores .
Safety and Hazards
Safety data sheets indicate that precautions should be taken to avoid dust formation, breathing mist, gas, or vapors, and contact with skin and eyes when handling 1-Ethyladamantane . Protective equipment, including chemical impermeable gloves, should be worn, and adequate ventilation should be ensured .
properties
IUPAC Name |
1-ethyladamantane | |
---|---|---|
Source | PubChem | |
URL | https://pubchem.ncbi.nlm.nih.gov | |
Description | Data deposited in or computed by PubChem | |
InChI |
InChI=1S/C12H20/c1-2-12-6-9-3-10(7-12)5-11(4-9)8-12/h9-11H,2-8H2,1H3 | |
Source | PubChem | |
URL | https://pubchem.ncbi.nlm.nih.gov | |
Description | Data deposited in or computed by PubChem | |
InChI Key |
LXTHCCWEYOKFSR-UHFFFAOYSA-N | |
Source | PubChem | |
URL | https://pubchem.ncbi.nlm.nih.gov | |
Description | Data deposited in or computed by PubChem | |
Canonical SMILES |
CCC12CC3CC(C1)CC(C3)C2 | |
Source | PubChem | |
URL | https://pubchem.ncbi.nlm.nih.gov | |
Description | Data deposited in or computed by PubChem | |
Molecular Formula |
C12H20 | |
Source | PubChem | |
URL | https://pubchem.ncbi.nlm.nih.gov | |
Description | Data deposited in or computed by PubChem | |
DSSTOX Substance ID |
DTXSID30335019 | |
Record name | 1-Ethyladamantane | |
Source | EPA DSSTox | |
URL | https://comptox.epa.gov/dashboard/DTXSID30335019 | |
Description | DSSTox provides a high quality public chemistry resource for supporting improved predictive toxicology. | |
Molecular Weight |
164.29 g/mol | |
Source | PubChem | |
URL | https://pubchem.ncbi.nlm.nih.gov | |
Description | Data deposited in or computed by PubChem | |
Product Name |
1-Ethyladamantane | |
CAS RN |
770-69-4 | |
Record name | 1-Ethyladamantane | |
Source | EPA DSSTox | |
URL | https://comptox.epa.gov/dashboard/DTXSID30335019 | |
Description | DSSTox provides a high quality public chemistry resource for supporting improved predictive toxicology. | |
Synthesis routes and methods I
Procedure details
Synthesis routes and methods II
Procedure details
Retrosynthesis Analysis
AI-Powered Synthesis Planning: Our tool employs the Template_relevance Pistachio, Template_relevance Bkms_metabolic, Template_relevance Pistachio_ringbreaker, Template_relevance Reaxys, Template_relevance Reaxys_biocatalysis model, leveraging a vast database of chemical reactions to predict feasible synthetic routes.
One-Step Synthesis Focus: Specifically designed for one-step synthesis, it provides concise and direct routes for your target compounds, streamlining the synthesis process.
Accurate Predictions: Utilizing the extensive PISTACHIO, BKMS_METABOLIC, PISTACHIO_RINGBREAKER, REAXYS, REAXYS_BIOCATALYSIS database, our tool offers high-accuracy predictions, reflecting the latest in chemical research and data.
Strategy Settings
Precursor scoring | Relevance Heuristic |
---|---|
Min. plausibility | 0.01 |
Model | Template_relevance |
Template Set | Pistachio/Bkms_metabolic/Pistachio_ringbreaker/Reaxys/Reaxys_biocatalysis |
Top-N result to add to graph | 6 |
Feasible Synthetic Routes
Q & A
Q1: What are the typical reaction pathways of 1-ethyladamantane during anodic oxidation?
A1: The anodic oxidation of 1-ethyladamantane can proceed through two main pathways: proton loss or fragmentation. In the presence of trifluoroacetic acid or acetonitrile, the initial step involves the formation of a charge-delocalized cation radical []. While simpler alkyladamantanes like 1-ethyladamantane primarily undergo deprotonation from the cation radical, bulkier substituents on the adamantane cage can shift the reaction towards fragmentation of a carbon-carbon bond []. This competition is governed by the stability of the resulting carbocation, with fragmentation being favored when a tertiary carbocation can be formed.
Q2: How does 1-ethyladamantane behave in binary mixtures with alcohols, and what insights do these mixtures provide about its potential applications?
A3: Studies on binary mixtures of 1-ethyladamantane with 1-heptanol and cyclohexylmethanol provide valuable insights into its physicochemical properties and potential applications []. Measurements of density, viscosity, refractive index, and surface tension across different temperatures and compositions reveal deviations from ideal behavior []. These deviations, quantified through excess molar volume, viscosity deviation, molar refraction deviation, and surface tension deviation, highlight the presence of molecular interactions between 1-ethyladamantane and the alcohols []. This data contributes to a fundamental understanding of adamantane derivatives as potential components in high energy-density hydrocarbon fuels [].
Q3: Has the catalytic oxidation of 1-ethyladamantane been investigated, and what are the key findings?
A4: The catalytic oxidation of 1-ethyladamantane has been studied using hydrogen peroxide as the oxidant and iron-pyridine complexes as catalysts []. This system utilizes atomic hydrogen generated by a palladium membrane to reduce iron in the catalytic complex []. Interestingly, oxidation occurs not only at the tertiary and secondary carbon atoms of the adamantane nucleus but also at the primary carbon atoms of the ethyl group []. Notably, alcohols are the major products of this reaction, exceeding the yield of ketones []. This selectivity towards alcohol formation highlights the potential for controlling the oxidation process through catalyst design and reaction conditions.
Q4: What analytical techniques are commonly employed to study 1-ethyladamantane and related compounds?
A6: Analyzing 1-ethyladamantane and similar compounds often relies on techniques such as gas chromatography-mass spectrometry (GC-MS). In the context of crude oil analysis, this method helps identify and quantify various diamondoids, including 1-ethyladamantane []. Researchers utilize specific mass-to-charge ratios (m/z) to identify different diamondoid species within complex mixtures []. For example, the presence of 1-ethyladamantane is typically indicated by a prominent peak at m/z 135 []. This analytical approach enables researchers to investigate the distribution and abundance of diamondoids in various samples, providing insights into geological processes and petroleum formation.
Disclaimer and Information on In-Vitro Research Products
Please be aware that all articles and product information presented on BenchChem are intended solely for informational purposes. The products available for purchase on BenchChem are specifically designed for in-vitro studies, which are conducted outside of living organisms. In-vitro studies, derived from the Latin term "in glass," involve experiments performed in controlled laboratory settings using cells or tissues. It is important to note that these products are not categorized as medicines or drugs, and they have not received approval from the FDA for the prevention, treatment, or cure of any medical condition, ailment, or disease. We must emphasize that any form of bodily introduction of these products into humans or animals is strictly prohibited by law. It is essential to adhere to these guidelines to ensure compliance with legal and ethical standards in research and experimentation.