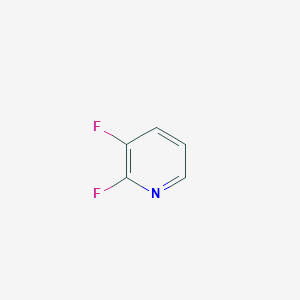
2,3-Difluoropyridine
Overview
Description
2,3-Difluoropyridine (2,3-DFPy, CAS 1513-66-2, C₅H₃F₂N, MW 115.08) is a fluorinated pyridine derivative with fluorine substituents at the 2- and 3-positions of the pyridine ring. Its electronic and structural properties make it a valuable intermediate in organic synthesis, particularly in metalation and cross-coupling reactions . High-resolution spectroscopic studies reveal an ionization energy of 9.6958 ± 0.0005 eV, attributed to its π and nN orbitals, with additional fluorine substitution at the α-position enhancing nN orbital stability compared to mono-fluorinated analogs . The compound is commercially available through global suppliers and serves as a precursor for pharmaceuticals and advanced materials.
Preparation Methods
Synthetic Routes and Reaction Conditions: The synthesis of 2,3-Difluoropyridine typically involves the fluorination of pyridine derivatives. One common method is the nucleophilic substitution reaction, where a chlorine atom in a chloropyridine derivative is replaced by a fluorine atom using a fluorinating agent such as potassium fluoride (KF) in a polar aprotic solvent like dimethylformamide (DMF). For example, 2,3,5-trichloropyridine can be reacted with potassium fluoride to yield this compound .
Industrial Production Methods: Industrial production of this compound often involves multi-step processes starting from readily available precursors. One such method involves the chlorination of 2-aminopyridine to produce 2-amino-3,5-dichloropyridine, followed by diazotization and Sandmeyer reaction to obtain 2,3,5-trichloropyridine. This intermediate is then fluorinated using a mixture of cesium fluoride and potassium fluoride in solvents like sulfolane and dimethyl sulfoxide at elevated temperatures .
Chemical Reactions Analysis
Types of Reactions: 2,3-Difluoropyridine undergoes various chemical reactions, including:
Nucleophilic Substitution: The fluorine atoms can be replaced by other nucleophiles under appropriate conditions.
Electrophilic Substitution: The compound can participate in electrophilic aromatic substitution reactions, although the presence of fluorine atoms makes the ring less reactive compared to non-fluorinated pyridines.
Reduction and Oxidation: It can undergo reduction and oxidation reactions, although these are less common.
Common Reagents and Conditions:
Nucleophilic Substitution: Potassium fluoride (KF) in dimethylformamide (DMF) or other polar aprotic solvents.
Electrophilic Substitution: Various electrophiles such as halogens, nitrating agents, and sulfonating agents under acidic or basic conditions.
Major Products Formed:
Substitution Products: Depending on the nucleophile used, various substituted pyridines can be formed.
Oxidation and Reduction Products: These reactions can lead to the formation of pyridine N-oxides or reduced pyridine derivatives.
Scientific Research Applications
Pharmaceutical Applications
Antiviral and Antimicrobial Agents:
2,3-Difluoropyridine serves as a building block in the synthesis of various pharmaceutical compounds. Its fluorine substituents enhance lipophilicity and metabolic stability, making it an attractive candidate for developing antiviral and antimicrobial agents. For instance, derivatives of difluoropyridine have shown promising activity against viral infections by modulating enzyme functions critical for viral replication .
Selective Functionalization:
Research has demonstrated that this compound can undergo regioselective functionalization, allowing for the introduction of diverse functional groups at specific positions on the pyridine ring. This property is particularly useful in medicinal chemistry for synthesizing targeted compounds with enhanced biological activity .
Materials Science
Polymer Chemistry:
The compound is utilized in the development of advanced materials, particularly fluorinated polymers. Due to its reactivity, this compound can be employed as a monomer or co-monomer in polymerization reactions to create materials with improved thermal stability and chemical resistance. These polymers find applications in coatings, adhesives, and aerospace materials where durability is paramount .
Fluorinated Coatings:
Fluorinated compounds like this compound are integral to formulating coatings that exhibit low surface energy and high resistance to solvents and chemicals. Such coatings are beneficial in industrial applications where protection against harsh environments is required .
Synthetic Chemistry
Reagent in Organic Synthesis:
In synthetic organic chemistry, this compound acts as a versatile reagent for various transformations. It can participate in nucleophilic substitutions and coupling reactions to form complex organic molecules efficiently. Its utility as a nucleophile or electrophile in synthetic pathways makes it a valuable compound for chemists .
Catalysis:
The compound has been explored as a ligand in transition metal-catalyzed reactions. Its ability to stabilize metal centers enhances catalytic activity and selectivity in cross-coupling reactions, which are vital for constructing carbon-carbon bonds in organic synthesis .
Case Studies
Mechanism of Action
The mechanism of action of 2,3-Difluoropyridine depends on its specific application. In biological systems, the fluorine atoms can influence the compound’s interaction with enzymes and receptors by altering electronic properties and steric factors. This can enhance binding affinity and selectivity for target molecules. For example, fluorinated pyridines have been studied as modulators of hepatocyte growth factor (HGF) pathways .
Comparison with Similar Compounds
Comparison with Similar Difluoropyridines
Structural Comparisons
Fluorine substitution patterns significantly influence bond lengths and molecular geometry (Table 1). Quantum chemical calculations show that 2,3-DFPy has a shorter average bond length (1.365 Å) compared to 3,5-difluoropyridine (1.3665 Å) but longer than 2,6-difluoropyridine (1.3622 Å) due to steric and electronic effects. The C–F bond lengths also vary, with 2,3-DFPy exhibiting shorter bonds (1.3385 Å) than 3-fluoropyridine (1.3482 Å), reflecting increased electron-withdrawing effects .
Table 1: Structural Parameters of Difluoropyridines
Compound | Avg. Bond Length (Å) | Avg. C–F Bond Length (Å) |
---|---|---|
Pyridine | 1.3709 | — |
2-Fluoropyridine | 1.3663 | 1.3443 |
2,3-Difluoropyridine | 1.3650 | 1.3385 |
2,6-Difluoropyridine | 1.3622 | 1.3387 |
3,5-Difluoropyridine | 1.3665 | 1.3449 |
Metalation and Cross-Coupling
2,3-DFPy undergoes selective lithiation at the 4-position, yielding regioisomerically pure products (67–90% yield) in Negishi cross-coupling reactions . However, its 3-fluorine resists nucleophilic aromatic substitution (SNAr), unlike 2,6- or 2,4-difluoropyridines. For example, attempts to synthesize benzofuropyridines from 2,3-DFPy failed, producing biaryl phenols instead .
Functionalization
Regioselective carboxylation of 2,3-DFPy is achievable using chlorine or trimethylsilyl groups as directing/protecting agents, enabling functionalization at all vacant positions . This contrasts with 2,5-difluoropyridine, where steric hindrance limits reactivity at the 4-position .
Electronic and Ionization Properties
2,3-DFPy’s ionization energy (9.6958 eV) reflects strong electron-withdrawing effects from fluorine substituents. Comparative studies with 2-fluoropyridine show that the additional fluorine at the α-position stabilizes the nN orbital, altering charge distribution in the π-system .
Table 2: Conductivity Properties of Difluoropyridine-Based Superconductors
Guest Molecule (G) | Conductivity Behavior | Transition Temperature |
---|---|---|
This compound | Metallic down to 1.4 K | None |
2,6-Difluoropyridine | Superconducting transition | 3.1 K |
Key Research Findings
- Synthetic Limitations : The 3-fluorine in 2,3-DFPy resists SNAr, limiting its use in benzofuropyridine synthesis .
- Regioselective Functionalization : Directed metalation enables precise functionalization, offering pathways to diverse derivatives .
- Material Design : Fluorine positioning critically impacts conductivity, with 2,6-DFPy favoring superconducting phases over 2,3-DFPy .
Biological Activity
2,3-Difluoropyridine is a fluorinated derivative of pyridine that has garnered attention due to its diverse biological activities and potential applications in medicinal chemistry. This article delves into the synthesis, biological evaluations, and structure-activity relationships (SAR) of this compound and its derivatives, highlighting key findings from recent studies.
Synthesis of this compound
The synthesis of this compound typically involves the fluorination of pyridine derivatives. One common method includes the diazotization-fluorination of aminopyridines in anhydrous hydrogen fluoride, which allows for selective introduction of fluorine atoms at the desired positions on the pyridine ring . The reaction conditions and choice of starting materials significantly influence the yield and purity of the final product.
Pharmacological Properties
Recent studies have highlighted the biological relevance of this compound in various pharmacological contexts:
- Dopamine Receptor Modulation : Compounds derived from this compound have been evaluated for their activity on dopamine D2 and D3 receptors. For instance, novel derivatives exhibited agonistic activity on these receptors with EC50 values ranging from 0.9 to 19 nmol/L for D2 and D3 receptors respectively . This suggests a potential role in treating disorders like Parkinson's disease through multitarget receptor engagement.
- Serotonin Receptor Activity : In addition to dopamine receptors, some derivatives also showed significant agonism at serotonin 5-HT1A receptors (EC50 values around 1.4 to 2.3 nmol/L), indicating a dual-action profile that could be beneficial for managing both motor and non-motor symptoms associated with Parkinson's disease .
Cytotoxicity Studies
The cytotoxic effects of 3-deaza-3-fluorouridine derivatives, which incorporate structural elements from difluoropyridines, were evaluated against various cancer cell lines. The compounds demonstrated cytostatic activity with CC(50) values ranging from 4.4 to 9.6 µM, indicating their potential as chemotherapeutic agents . However, no significant antiviral activity was observed against virus-infected cell cultures.
Structure-Activity Relationship (SAR)
The SAR analysis of this compound derivatives indicates that modifications at specific positions on the pyridine ring can enhance biological activity:
Substituent Position | Modification | Effect on Activity |
---|---|---|
2 | Fluorine | Increased potency for D2/D3 receptor agonism |
5 | Chlorine or Bromine | Varied effects; some decrease activity significantly |
4 | Alkyl or Aryl groups | Generally increases receptor interaction |
This table summarizes how different substituents influence the pharmacological properties of difluoropyridine derivatives, emphasizing the importance of strategic modifications in drug design.
Case Studies
- Multitarget Agonists for Parkinson's Disease : A study synthesized a series of pyridyl alkylarylpiperazine derivatives based on this compound. The lead compound showed promising multitarget activity against D2/D3 and 5-HT1A receptors, suggesting its potential as a therapeutic agent for Parkinson's disease .
- Cancer Cell Line Evaluations : The cytotoxicity of various difluoropyridine derivatives was tested across multiple cancer cell lines. The results indicated that certain modifications could enhance selectivity and potency against tumor cells while maintaining low toxicity to normal cells .
Q & A
Basic Research Questions
Q. What methods are commonly used to synthesize 2,3-difluoropyridine, and how can purity be optimized?
Answer: this compound is typically synthesized via halogen exchange reactions or selective fluorination of pyridine precursors. For example, halogenated intermediates like 2,3-dichloropyridine can undergo fluorination using potassium fluoride (KF) or hydrogen fluoride (HF) under controlled conditions. A study by Bobbio and Schlosser (2005) demonstrated selective functionalization at vacant positions of fluoropyridines using organometallic intermediates, which can be adapted for regioselective synthesis . Purity optimization involves chromatographic techniques (e.g., HPLC or column chromatography) and recrystallization. Analytical methods such as GC-MS or NMR spectroscopy are critical for verifying purity (>97% as per commercial standards) .
Q. How is the molecular structure of this compound determined experimentally?
Answer: Microwave spectroscopy and rotational constants are key for structural determination. For instance, microwave studies on 2,6-difluoropyridine (a structural analog) revealed bond lengths and angles through isotopic substitution (e.g., and isotopes) and double-resonance modulated (DRM) spectroscopy . For this compound, similar methods can resolve its planar geometry and substituent effects. Structural parameters like χaa (1.658 MHz) and χbb (-4.598 MHz) derived from microwave spectra provide insights into its quadrupole coupling constants and ring distortion .
Advanced Research Questions
Q. What are the electronic properties and ionization dynamics of this compound?
Answer: High-resolution vacuum ultraviolet mass-analyzed threshold ionization (VUV-MATI) spectroscopy reveals an ionization energy of 9.6958 ± 0.0005 eV for this compound. Upon ionization, the molecule undergoes out-of-plane ring distortion, transitioning from to symmetry. Quantum chemical calculations (e.g., TD-DFT) identify the highest occupied molecular orbital (HOMO) as the π orbital and HOMO-1 as the nitrogen lone pair (nN). Fluorine substitution at the α-position destabilizes the nN orbital compared to monofluorinated analogs, impacting reactivity .
Q. How do fluorine substituents influence the vibrational modes and reactivity of this compound?
Answer: Vibrational spectroscopy (e.g., NEXAFS) shows that fluorine substitution alters the C–F stretching and ring deformation modes. For example, in 2,6-difluoropyridine, fluorination shifts the C1s→π* transition energy, indicating electronic redistribution . Reactivity studies reveal that fluorine atoms direct nucleophilic substitution to specific positions. For this compound, the 4-position is more reactive due to reduced steric hindrance and electronic effects, as shown in regioselective amination and cross-coupling reactions .
Q. What challenges arise in synthesizing derivatives of this compound with additional functional groups?
Answer: Introducing multiple functional groups (e.g., cyano, amino) requires overcoming steric and electronic barriers. For example, 2,6-difluoro-3-cyanopyridine synthesis demands precise temperature control to avoid side reactions. Optimizing catalysts (e.g., palladium for cross-coupling) and protecting groups (e.g., tert-butoxycarbonyl for amines) is critical. Contradictions in reported yields (e.g., 60–85%) highlight the need for protocol standardization, including solvent selection (DMF vs. THF) and reaction time .
Q. How can spectroscopic data resolve contradictions in reported structural parameters of this compound?
Answer: Discrepancies in quadrupole coupling constants (e.g., χaa = 1.658 MHz vs. 2.644 MHz for Ar-complexed forms) may arise from experimental conditions (e.g., gas-phase vs. matrix isolation). Combining microwave spectroscopy with computational methods (e.g., MP2 or CCSD(T)) validates structural parameters. For instance, DRM spectroscopy confirmed bond lengths within 0.01 Å accuracy for 2,6-difluoropyridine, a strategy applicable to this compound .
Q. Methodological Guidelines
- Synthesis: Prioritize halogen exchange with KF in polar aprotic solvents (e.g., DMF) at 150–200°C .
- Structural Analysis: Use DRM microwave spectroscopy for isotopic substitution studies and DFT for vibrational mode assignments .
- Electronic Studies: Pair VUV-MATI spectroscopy with NBO analysis to map orbital interactions .
- Reactivity Screening: Employ directed ortho-metalation (DoM) for regioselective functionalization .
Properties
IUPAC Name |
2,3-difluoropyridine | |
---|---|---|
Source | PubChem | |
URL | https://pubchem.ncbi.nlm.nih.gov | |
Description | Data deposited in or computed by PubChem | |
InChI |
InChI=1S/C5H3F2N/c6-4-2-1-3-8-5(4)7/h1-3H | |
Source | PubChem | |
URL | https://pubchem.ncbi.nlm.nih.gov | |
Description | Data deposited in or computed by PubChem | |
InChI Key |
OGVLEPMNNPZAPS-UHFFFAOYSA-N | |
Source | PubChem | |
URL | https://pubchem.ncbi.nlm.nih.gov | |
Description | Data deposited in or computed by PubChem | |
Canonical SMILES |
C1=CC(=C(N=C1)F)F | |
Source | PubChem | |
URL | https://pubchem.ncbi.nlm.nih.gov | |
Description | Data deposited in or computed by PubChem | |
Molecular Formula |
C5H3F2N | |
Source | PubChem | |
URL | https://pubchem.ncbi.nlm.nih.gov | |
Description | Data deposited in or computed by PubChem | |
DSSTOX Substance ID |
DTXSID70382486 | |
Record name | 2,3-Difluoropyridine | |
Source | EPA DSSTox | |
URL | https://comptox.epa.gov/dashboard/DTXSID70382486 | |
Description | DSSTox provides a high quality public chemistry resource for supporting improved predictive toxicology. | |
Molecular Weight |
115.08 g/mol | |
Source | PubChem | |
URL | https://pubchem.ncbi.nlm.nih.gov | |
Description | Data deposited in or computed by PubChem | |
CAS No. |
1513-66-2 | |
Record name | 2,3-Difluoropyridine | |
Source | CAS Common Chemistry | |
URL | https://commonchemistry.cas.org/detail?cas_rn=1513-66-2 | |
Description | CAS Common Chemistry is an open community resource for accessing chemical information. Nearly 500,000 chemical substances from CAS REGISTRY cover areas of community interest, including common and frequently regulated chemicals, and those relevant to high school and undergraduate chemistry classes. This chemical information, curated by our expert scientists, is provided in alignment with our mission as a division of the American Chemical Society. | |
Explanation | The data from CAS Common Chemistry is provided under a CC-BY-NC 4.0 license, unless otherwise stated. | |
Record name | 2,3-Difluoropyridine | |
Source | EPA DSSTox | |
URL | https://comptox.epa.gov/dashboard/DTXSID70382486 | |
Description | DSSTox provides a high quality public chemistry resource for supporting improved predictive toxicology. | |
Record name | 2,3-Difluoropyridine | |
Source | European Chemicals Agency (ECHA) | |
URL | https://echa.europa.eu/information-on-chemicals | |
Description | The European Chemicals Agency (ECHA) is an agency of the European Union which is the driving force among regulatory authorities in implementing the EU's groundbreaking chemicals legislation for the benefit of human health and the environment as well as for innovation and competitiveness. | |
Explanation | Use of the information, documents and data from the ECHA website is subject to the terms and conditions of this Legal Notice, and subject to other binding limitations provided for under applicable law, the information, documents and data made available on the ECHA website may be reproduced, distributed and/or used, totally or in part, for non-commercial purposes provided that ECHA is acknowledged as the source: "Source: European Chemicals Agency, http://echa.europa.eu/". Such acknowledgement must be included in each copy of the material. ECHA permits and encourages organisations and individuals to create links to the ECHA website under the following cumulative conditions: Links can only be made to webpages that provide a link to the Legal Notice page. | |
Retrosynthesis Analysis
AI-Powered Synthesis Planning: Our tool employs the Template_relevance Pistachio, Template_relevance Bkms_metabolic, Template_relevance Pistachio_ringbreaker, Template_relevance Reaxys, Template_relevance Reaxys_biocatalysis model, leveraging a vast database of chemical reactions to predict feasible synthetic routes.
One-Step Synthesis Focus: Specifically designed for one-step synthesis, it provides concise and direct routes for your target compounds, streamlining the synthesis process.
Accurate Predictions: Utilizing the extensive PISTACHIO, BKMS_METABOLIC, PISTACHIO_RINGBREAKER, REAXYS, REAXYS_BIOCATALYSIS database, our tool offers high-accuracy predictions, reflecting the latest in chemical research and data.
Strategy Settings
Precursor scoring | Relevance Heuristic |
---|---|
Min. plausibility | 0.01 |
Model | Template_relevance |
Template Set | Pistachio/Bkms_metabolic/Pistachio_ringbreaker/Reaxys/Reaxys_biocatalysis |
Top-N result to add to graph | 6 |
Feasible Synthetic Routes
Disclaimer and Information on In-Vitro Research Products
Please be aware that all articles and product information presented on BenchChem are intended solely for informational purposes. The products available for purchase on BenchChem are specifically designed for in-vitro studies, which are conducted outside of living organisms. In-vitro studies, derived from the Latin term "in glass," involve experiments performed in controlled laboratory settings using cells or tissues. It is important to note that these products are not categorized as medicines or drugs, and they have not received approval from the FDA for the prevention, treatment, or cure of any medical condition, ailment, or disease. We must emphasize that any form of bodily introduction of these products into humans or animals is strictly prohibited by law. It is essential to adhere to these guidelines to ensure compliance with legal and ethical standards in research and experimentation.