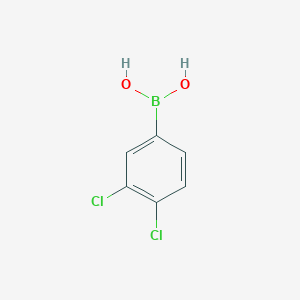
3,4-Dichlorophenylboronic acid
Overview
Description
3,4-Dichlorophenylboronic acid is an organoboron compound with the chemical formula C6H5BCl2O2. It is widely used in organic synthesis and medicinal chemistry due to its ability to form carbon-carbon bonds through Suzuki coupling reactions . This compound is characterized by the presence of two chlorine atoms attached to the benzene ring, which significantly influences its reactivity and applications.
Mechanism of Action
Target of Action
3,4-Dichlorophenylboronic acid is a reactant used in the synthesis of various biologically active molecules . It has been involved in the synthesis of compounds such as Mycobacterium tuberculosis H37Rv chorismate mutase inhibitors .
Mode of Action
The compound interacts with its targets through chemical reactions. For instance, it is involved in Suzuki coupling reactions . This is a type of palladium-catalyzed cross coupling reaction that allows for the formation of carbon-carbon bonds.
Biochemical Pathways
The compound affects various biochemical pathways. For instance, it is involved in the lithiation/borylation-protodeboronation of homoallyl carbamates . This process is part of the synthesis of various biologically active molecules.
Result of Action
The result of the action of this compound is the synthesis of various biologically active molecules. For instance, it has been used in the synthesis of pyrrole derivatives as PDE4B inhibitors . These inhibitors can have potential therapeutic applications in various diseases.
Action Environment
The action of this compound can be influenced by various environmental factors. For instance, the compound should be stored in a dark place, sealed in dry, and at room temperature . This helps to maintain its stability and efficacy.
Biochemical Analysis
Biochemical Properties
3,4-Dichlorophenylboronic acid plays a significant role in various biochemical reactions. It is known to interact with enzymes such as Mycobacterium tuberculosis H37Rv chorismate mutase inhibitors and pyrrole derivatives as PDE4B inhibitors . These interactions are crucial for the synthesis of biologically active molecules, including anticancer agents and dual immunosuppressive and anti-inflammatory agents . The compound’s ability to form stable complexes with these enzymes and proteins highlights its importance in biochemical research.
Cellular Effects
The effects of this compound on cellular processes are profound. It influences cell function by modulating cell signaling pathways, gene expression, and cellular metabolism. For instance, it has been observed to affect the glycan domain of antibodies, which plays a critical role in immune response . Additionally, its interaction with various cellular proteins can lead to changes in cellular metabolism, impacting overall cell health and function.
Molecular Mechanism
At the molecular level, this compound exerts its effects through specific binding interactions with biomolecules. It acts as an enzyme inhibitor in several biochemical pathways, including the inhibition of chorismate mutase and PDE4B . These interactions result in changes in gene expression and enzyme activity, ultimately affecting cellular processes. The compound’s ability to form boronate esters with diols is a key aspect of its molecular mechanism, enabling it to interact with a wide range of biomolecules.
Temporal Effects in Laboratory Settings
In laboratory settings, the effects of this compound can vary over time. The compound is relatively stable under standard conditions, but its stability can be influenced by factors such as temperature and pH . Over time, degradation products may form, which can impact its efficacy in biochemical assays. Long-term studies have shown that the compound can have sustained effects on cellular function, although the specific outcomes depend on the experimental conditions.
Dosage Effects in Animal Models
The effects of this compound in animal models are dose-dependent. At lower doses, the compound may exhibit beneficial effects, such as anti-inflammatory and immunosuppressive properties . At higher doses, toxic or adverse effects can occur, including potential damage to vital organs and disruption of normal cellular processes. It is crucial to determine the optimal dosage to maximize therapeutic benefits while minimizing adverse effects.
Metabolic Pathways
This compound is involved in several metabolic pathways. It interacts with enzymes and cofactors that facilitate its conversion into various metabolites . These metabolic transformations can affect the compound’s bioavailability and activity. Additionally, the compound’s impact on metabolic flux and metabolite levels can provide insights into its potential therapeutic applications.
Transport and Distribution
Within cells and tissues, this compound is transported and distributed through specific transporters and binding proteins . These interactions influence its localization and accumulation in different cellular compartments. Understanding the transport mechanisms is essential for optimizing the compound’s delivery and efficacy in therapeutic applications.
Subcellular Localization
The subcellular localization of this compound is determined by targeting signals and post-translational modifications . These factors direct the compound to specific compartments or organelles, where it can exert its biochemical effects. The localization can impact the compound’s activity and function, making it a critical aspect of its overall mechanism of action.
Preparation Methods
Synthetic Routes and Reaction Conditions: The synthesis of 3,4-Dichlorophenylboronic acid typically involves the reaction of 3,4-dichlorophenylmagnesium bromide with trimethyl borate, followed by hydrolysis. The reaction conditions often include:
Solvent: Tetrahydrofuran (THF)
Temperature: -78°C to room temperature
Reagents: 3,4-dichlorophenylmagnesium bromide, trimethyl borate, water
Industrial Production Methods: In industrial settings, the production of this compound may involve more scalable processes, such as:
Continuous flow synthesis: This method allows for better control over reaction conditions and improved safety.
Catalytic processes: Utilizing catalysts to enhance the efficiency and yield of the reaction.
Chemical Reactions Analysis
Types of Reactions: 3,4-Dichlorophenylboronic acid undergoes various chemical reactions, including:
Suzuki Coupling Reactions: This is the most common reaction, where it reacts with aryl or vinyl halides in the presence of a palladium catalyst to form biaryl compounds.
Oxidation: It can be oxidized to form phenols.
Substitution: The chlorine atoms can be substituted with other functional groups under appropriate conditions.
Common Reagents and Conditions:
Suzuki Coupling: Palladium catalyst, base (e.g., potassium carbonate), solvent (e.g., toluene or ethanol), temperature (80-100°C).
Oxidation: Oxidizing agents such as hydrogen peroxide or potassium permanganate.
Substitution: Nucleophiles such as amines or thiols.
Major Products:
Biaryl Compounds: Formed through Suzuki coupling.
Phenols: Formed through oxidation.
Substituted Derivatives: Formed through substitution reactions.
Scientific Research Applications
3,4-Dichlorophenylboronic acid has a wide range of applications in scientific research, including:
Chemistry: Used as a building block in the synthesis of complex organic molecules, particularly in the formation of carbon-carbon bonds.
Biology: Employed in the development of enzyme inhibitors and probes for biological studies.
Medicine: Utilized in the synthesis of pharmaceutical compounds, including potential anticancer agents and enzyme inhibitors.
Industry: Applied in the production of advanced materials and polymers.
Comparison with Similar Compounds
- 2,4-Dichlorophenylboronic acid
- 4-Chlorophenylboronic acid
- 3-Chlorophenylboronic acid
- Phenylboronic acid
Comparison: 3,4-Dichlorophenylboronic acid is unique due to the presence of two chlorine atoms on the benzene ring, which enhances its reactivity and selectivity in certain reactions. Compared to phenylboronic acid, the dichloro derivative exhibits different electronic properties, making it more suitable for specific applications in organic synthesis and medicinal chemistry.
Properties
IUPAC Name |
(3,4-dichlorophenyl)boronic acid | |
---|---|---|
Source | PubChem | |
URL | https://pubchem.ncbi.nlm.nih.gov | |
Description | Data deposited in or computed by PubChem | |
InChI |
InChI=1S/C6H5BCl2O2/c8-5-2-1-4(7(10)11)3-6(5)9/h1-3,10-11H | |
Source | PubChem | |
URL | https://pubchem.ncbi.nlm.nih.gov | |
Description | Data deposited in or computed by PubChem | |
InChI Key |
JKIGHOARKAIPJI-UHFFFAOYSA-N | |
Source | PubChem | |
URL | https://pubchem.ncbi.nlm.nih.gov | |
Description | Data deposited in or computed by PubChem | |
Canonical SMILES |
B(C1=CC(=C(C=C1)Cl)Cl)(O)O | |
Source | PubChem | |
URL | https://pubchem.ncbi.nlm.nih.gov | |
Description | Data deposited in or computed by PubChem | |
Molecular Formula |
C6H5BCl2O2 | |
Source | PubChem | |
URL | https://pubchem.ncbi.nlm.nih.gov | |
Description | Data deposited in or computed by PubChem | |
DSSTOX Substance ID |
DTXSID10370221 | |
Record name | 3,4-Dichlorophenylboronic acid | |
Source | EPA DSSTox | |
URL | https://comptox.epa.gov/dashboard/DTXSID10370221 | |
Description | DSSTox provides a high quality public chemistry resource for supporting improved predictive toxicology. | |
Molecular Weight |
190.82 g/mol | |
Source | PubChem | |
URL | https://pubchem.ncbi.nlm.nih.gov | |
Description | Data deposited in or computed by PubChem | |
CAS No. |
151169-75-4 | |
Record name | 3,4-Dichlorophenylboronic acid | |
Source | EPA DSSTox | |
URL | https://comptox.epa.gov/dashboard/DTXSID10370221 | |
Description | DSSTox provides a high quality public chemistry resource for supporting improved predictive toxicology. | |
Record name | 3,4-Dichlorobenzeneboronic acid | |
Source | European Chemicals Agency (ECHA) | |
URL | https://echa.europa.eu/information-on-chemicals | |
Description | The European Chemicals Agency (ECHA) is an agency of the European Union which is the driving force among regulatory authorities in implementing the EU's groundbreaking chemicals legislation for the benefit of human health and the environment as well as for innovation and competitiveness. | |
Explanation | Use of the information, documents and data from the ECHA website is subject to the terms and conditions of this Legal Notice, and subject to other binding limitations provided for under applicable law, the information, documents and data made available on the ECHA website may be reproduced, distributed and/or used, totally or in part, for non-commercial purposes provided that ECHA is acknowledged as the source: "Source: European Chemicals Agency, http://echa.europa.eu/". Such acknowledgement must be included in each copy of the material. ECHA permits and encourages organisations and individuals to create links to the ECHA website under the following cumulative conditions: Links can only be made to webpages that provide a link to the Legal Notice page. | |
Retrosynthesis Analysis
AI-Powered Synthesis Planning: Our tool employs the Template_relevance Pistachio, Template_relevance Bkms_metabolic, Template_relevance Pistachio_ringbreaker, Template_relevance Reaxys, Template_relevance Reaxys_biocatalysis model, leveraging a vast database of chemical reactions to predict feasible synthetic routes.
One-Step Synthesis Focus: Specifically designed for one-step synthesis, it provides concise and direct routes for your target compounds, streamlining the synthesis process.
Accurate Predictions: Utilizing the extensive PISTACHIO, BKMS_METABOLIC, PISTACHIO_RINGBREAKER, REAXYS, REAXYS_BIOCATALYSIS database, our tool offers high-accuracy predictions, reflecting the latest in chemical research and data.
Strategy Settings
Precursor scoring | Relevance Heuristic |
---|---|
Min. plausibility | 0.01 |
Model | Template_relevance |
Template Set | Pistachio/Bkms_metabolic/Pistachio_ringbreaker/Reaxys/Reaxys_biocatalysis |
Top-N result to add to graph | 6 |
Feasible Synthetic Routes
Q1: What spectroscopic data is available for 3,4-Dichlorophenylboronic acid, and what structural information can be derived from it?
A1: The provided research [] focuses on analyzing the infrared (IR) and Raman spectra of this compound. These techniques provide valuable insights into the molecule's structure:
Disclaimer and Information on In-Vitro Research Products
Please be aware that all articles and product information presented on BenchChem are intended solely for informational purposes. The products available for purchase on BenchChem are specifically designed for in-vitro studies, which are conducted outside of living organisms. In-vitro studies, derived from the Latin term "in glass," involve experiments performed in controlled laboratory settings using cells or tissues. It is important to note that these products are not categorized as medicines or drugs, and they have not received approval from the FDA for the prevention, treatment, or cure of any medical condition, ailment, or disease. We must emphasize that any form of bodily introduction of these products into humans or animals is strictly prohibited by law. It is essential to adhere to these guidelines to ensure compliance with legal and ethical standards in research and experimentation.