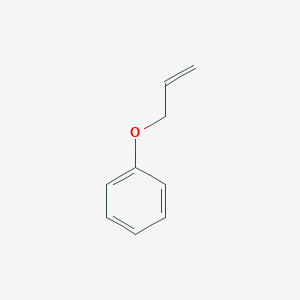
Allyl phenyl ether
Overview
Description
Allyl phenyl ether (CAS 1746-13-0) is an aromatic ether characterized by an oxygen atom bridging a phenyl group and an allyl (CH₂=CHCH₂–) moiety. It is a versatile compound in organic synthesis, notably serving as the classic substrate for the Claisen rearrangement—a thermally or photochemically induced [3,3]-sigmatropic rearrangement yielding o-allylphenol (Scheme 1) . Structural studies using microwave spectroscopy (8–14 GHz) have elucidated its planar geometry and rotational constants, critical for understanding its reactivity . Industrially, it is employed in agrochemical synthesis and as a precursor for functionalized aromatic compounds .
Preparation Methods
Williamson Ether Synthesis: Foundation of Allyl Phenyl Ether Preparation
The Williamson ether synthesis remains the cornerstone of this compound production, leveraging the reaction between an alkoxide ion and a primary alkyl halide. This method’s versatility allows adaptation across academic and industrial settings.
Mechanistic Overview
The reaction proceeds via an Sₙ2 mechanism: the phenoxide ion (C₆H₅O⁻) nucleophilically attacks the electrophilic carbon in allyl halides (CH₂=CH-CH₂-X, X = Br, Cl), displacing the halide ion. The choice of halide and reaction conditions critically influences yield and purity .
Traditional Protocol: Allyl Bromide and Sodium Phenoxide
In academic laboratories, allyl bromide (CH₂=CH-CH₂Br) reacts with sodium phenoxide (C₆H₅ONa) in polar solvents like ethanol or isopropanol. A typical procedure involves:
-
Dissolving phenol in a water-miscible solvent (e.g., isopropanol).
-
Adding sodium hydroxide to generate sodium phenoxide in situ.
-
Introducing allyl bromide under reflux (70–80°C) for 3–5 hours .
Key Data:
Parameter | Value | Source |
---|---|---|
Solvent | Isopropanol/water | |
Temperature | 70°C | |
Reaction Time | 5 hours | |
Purification | Water washes, distillation |
This method achieves high yields (>80%) but faces challenges in handling volatile allyl bromide, necessitating stringent safety measures .
Allyl Chloride Variation
Industrial processes often substitute allyl bromide with cheaper allyl chloride (CH₂=CH-CH₂Cl), albeit with modified conditions:
-
Higher temperatures (70–100°C) to overcome lower reactivity.
-
Autogenous pressure systems to prevent chloride evaporation.
A patent by details a scalable approach:
-
Combine phenol (8.0 mol), isopropanol (1.2 L), and NaOH (8.0 mol).
-
Add allyl chloride (9.6 mol) under nitrogen pressure (70 kPa).
-
React at 70°C for 5 hours, followed by toluene-assisted azeotropic distillation .
Industrial-Scale Synthesis: Optimizing Yield and Purity
Industrial protocols enhance the Williamson synthesis by integrating in situ alkoxide generation and advanced purification, addressing scalability and cost-efficiency.
In Situ Sodium Phenoxide Formation
Eliminating pre-formed alkoxide, manufacturers directly react phenol with NaOH in polar solvents (e.g., isopropanol). This one-pot method reduces handling of corrosive phenoxide powders .
-
Dissolve phenol (753 g) in isopropanol (1.2 L).
-
Add NaOH (320 g) in water (300 mL) to form sodium phenoxide.
-
Introduce allyl chloride (9.6 mol) at 70°C for 5 hours.
Azeotropic Purification
Post-reaction mixtures contain by-products (unreacted phenol, NaCl, solvents). Industrial setups employ non-polar solvents (e.g., toluene) for azeotropic distillation:
-
Add toluene to the crude mixture.
-
Heat to 100°C, distilling off water, isopropanol, and residual allyl chloride .
-
Retain pure this compound for subsequent use.
Advantages:
-
Eliminates energy-intensive vacuum distillation.
Alternative Synthesis Approaches
Simultaneous Reactant Addition
Japanese Patent JPH08333293A discloses a method where allyl halide and aqueous NaOH are simultaneously dripped into phenol:
-
Dissolve allyl chloride in molten phenol (40–100°C).
-
Gradually add 50% NaOH solution over 1–3 hours.
-
Maintain reaction at 80°C for 2–5 hours.
Benefits:
-
Minimizes side reactions (e.g., hydrolysis of allyl halide).
-
Yields 86–92% this compound after acid washing and dehydration .
Expanding Substrate Scope
Recent efforts explore diverse phenols (e.g., naphthols, bisphenols) and allylating agents:
-
Allyl iodide: Faster reactivity but cost-prohibitive.
-
Substituted allyl bromides: Introduce functional groups (e.g., CH₂=CH-CH₂-O-C₆H₄-CH₃) .
Comparative Analysis of Methods
Method | Reagents | Conditions | Yield | Scalability |
---|---|---|---|---|
Classic Williamson | Allyl Br + NaOPh | 70°C, 5 hrs | 80–85% | Moderate |
Industrial (Allyl Cl) | Allyl Cl + NaOH | 70–100°C, 5–10 hrs | 75–80% | High |
Simultaneous Addition | Allyl Cl + NaOH | 80°C, 3 hrs | 86–92% | High |
Key Findings:
-
Allyl chloride methods favor cost-efficiency but require longer reactions.
-
Simultaneous addition optimizes yield and reduces by-products .
-
Academic protocols prioritize simplicity, while industrial methods emphasize purification .
Experimental Case Studies
Academic Laboratory Procedure ( )
-
Alkoxide Formation: Add NaOH (0.1 mol) to phenol (0.1 mol) in ethanol.
-
Alkylation: Introduce allyl bromide (0.12 mol), reflux for 4 hours.
-
Workup: Extract with diethyl ether, wash with brine, dry over MgSO₄.
-
Yield: 78% after vacuum distillation.
Industrial Pilot-Scale Synthesis ( )
-
React 8.0 mol phenol with 8.0 mol NaOH in isopropanol/water.
-
Add 9.6 mol allyl chloride under N₂ at 70°C for 5 hours.
-
Purify via toluene azeotrope (100°C, 3 hours).
-
Output: 7.2 mol this compound (90% yield based on phenol).
Chemical Reactions Analysis
Types of Reactions: Allyl phenyl ether undergoes various chemical reactions, including:
Claisen Rearrangement: In the presence of acid catalysts, this compound converts to 2-allylphenol through a [3,3]-sigmatropic rearrangement.
Oxidation: this compound can be oxidized to form corresponding epoxides or aldehydes under specific conditions.
Substitution: The allyl group can participate in nucleophilic substitution reactions, forming different substituted phenyl ethers.
Common Reagents and Conditions:
Acid Catalysts: Used in Claisen rearrangement to convert this compound to 2-allylphenol.
Oxidizing Agents: Such as potassium permanganate or chromium trioxide for oxidation reactions.
Nucleophiles: For substitution reactions, common nucleophiles include halides and amines.
Major Products:
2-Allylphenol: Formed through Claisen rearrangement.
Epoxides and Aldehydes: Formed through oxidation reactions.
Substituted Phenyl Ethers: Formed through nucleophilic substitution.
Scientific Research Applications
Allyl phenyl ether has diverse applications in scientific research:
Chemistry: Used as an intermediate in the synthesis of various organic compounds, including pharmaceuticals and agrochemicals.
Biology: Employed in studies involving enzyme-catalyzed reactions and metabolic pathways.
Medicine: Investigated for its potential therapeutic properties and as a precursor in drug synthesis.
Industry: Utilized in the production of polymers, resins, and other industrial chemicals
Mechanism of Action
The primary mechanism of action for allyl phenyl ether involves the Claisen rearrangement. This reaction proceeds through a concerted mechanism where the C-O bond of the ether breaks, and a new C-C bond forms between the allyl group and the ortho position of the benzene ring . The reaction is characterized by the migration of a double bond and the formation of a new double bond, leading to the formation of 2-allylphenol.
Comparison with Similar Compounds
Reactivity in Transition-Metal-Free Allylation
Allyl phenyl ether exhibits superior reactivity compared to other allyl ethers in transition-metal-free allylation reactions. For example, in the synthesis of homoallylic amines, this compound achieved an 89% atom yield (AY), significantly outperforming allyl methyl ether (18% AY), allyl benzyl ether (14% AY), and allyl acetate (19% AY) (Table 1) . The electron-withdrawing phenyl group likely stabilizes transition states, enhancing leaving-group displacement efficiency.
Claisen Rearrangement Efficiency
This compound is the benchmark substrate for Claisen rearrangements due to its balanced electronic and steric properties. In contrast, allyl ethers with bulky substituents (e.g., allyl tert-butyl silyl ether, 16% AY) or electron-donating groups (e.g., allyl methyl ether) show reduced rearrangement efficiency . Photochemical Claisen rearrangements further highlight its adaptability, as competing processes (e.g., C–O bond cleavage) are minimized compared to benzyl phenyl ether .
Industrial and Market Relevance
Global market analyses (2019–2024) rank this compound as a high-demand compound in agrochemical and pharmaceutical sectors, with production volumes exceeding those of niche allyl ethers (e.g., allyl guaiacyl ether) . Its commercial viability is attributed to scalable synthesis and diverse applications.
Environmental and Green Chemistry
This compound participates in zeolite-mediated isomerization and photo-Claisen rearrangements under mild conditions, reducing reliance on hazardous solvents . Comparatively, allyl glycidyl ether requires harsher conditions (e.g., 245°C) for epoxide transformations, limiting its green chemistry applications .
Biological Activity
Allyl phenyl ether (APE) is an organic compound with a significant interest in various fields, particularly in organic synthesis and potential biological applications. This article explores the biological activity of APE, including its mechanisms, effects on different biological systems, and relevant case studies.
Chemical Structure and Properties
This compound has the molecular formula C10H10O and is characterized by an allyl group (C3H5) attached to a phenyl ether structure. Its chemical structure can be represented as follows:
Biological Activity Overview
The biological activity of this compound has been studied in various contexts, including its reactivity under different conditions and its potential therapeutic applications. The following sections summarize key findings from research studies.
Antimicrobial Activity
Research indicates that this compound exhibits antimicrobial properties. In a study evaluating various allylic compounds, APE demonstrated significant activity against several bacterial strains. The mechanism of action appears to involve disruption of microbial cell membranes, leading to cell lysis and death.
Antitumor Effects
This compound has also been investigated for its antitumor properties. In vitro studies showed that APE can induce apoptosis in cancer cell lines, such as MCF-7 (breast cancer) and HCT-116 (colon cancer). The proposed mechanisms include the activation of caspases and modulation of Bcl-2 family proteins, leading to increased Bax/Bcl-2 ratios and subsequent mitochondrial dysfunction.
Case Studies
-
Study on Antimicrobial Activity :
- APE was tested against Staphylococcus aureus and Escherichia coli .
- Results indicated a minimum inhibitory concentration (MIC) of 50 µg/mL for both bacteria.
- The study concluded that APE could be a potential candidate for developing new antimicrobial agents.
-
Antitumor Activity in Breast Cancer Cells :
- In a controlled experiment, MCF-7 cells were treated with varying concentrations of APE.
- Results showed a dose-dependent decrease in cell viability, with IC50 values determined at 25 µM after 48 hours.
- Mechanistic studies revealed that APE treatment led to increased levels of reactive oxygen species (ROS), contributing to oxidative stress-induced apoptosis.
Reaction Mechanisms
The Claisen rearrangement of this compound has been studied extensively to understand its reactivity under high-temperature conditions. Research shows that heating APE in water at temperatures above 180 °C leads to the formation of various products, including 2-allylphenol and 2-methyl-2,3-dihydrobenzofuran. The reaction pathway involves bond breaking and formation processes, which are critical for understanding its biological interactions.
Temperature (°C) | Product Yield (%) | Main Product |
---|---|---|
180 | 30 | 2-allylphenol |
200 | 56 | 2-allylphenol |
250 | 72 | 2-methyl-2,3-dihydrobenzofuran |
Q & A
Basic Research Questions
Q. What are the standard synthetic routes for preparing allyl phenyl ether, and how can purity be optimized?
this compound is typically synthesized via nucleophilic substitution using sodium phenoxide and allyl bromide under controlled heating (80–100°C). Critical steps include:
- Reagent stoichiometry : A 1:1 molar ratio of sodium phenoxide to allyl bromide minimizes side products like dialkylated ethers .
- Solvent selection : Anhydrous ethanol or THF improves yield by reducing hydrolysis of the allyl bromide .
- Purification : Distillation under reduced pressure (b.p. 76–78°C at 12 mmHg) followed by GC-MS analysis ensures >99% purity .
Q. What experimental techniques are used to confirm the Claisen rearrangement of this compound?
The Claisen rearrangement (conversion to o-allylphenol) is monitored using:
- NMR spectroscopy : Disappearance of the allyl ether’s vinyl protons (δ 5.8–6.2 ppm) and emergence of o-allylphenol aromatic signals (δ 6.6–7.4 ppm) .
- Kinetic analysis : First-order rate constants are derived via HPLC or inline NIR spectroscopy under controlled temperatures (180–280°C) .
- Isotopic labeling : -labeled this compound tracks carbon migration during rearrangement .
Advanced Research Questions
Q. How can discrepancies between theoretical and experimental kinetic isotope effects (KIEs) in Claisen rearrangements be resolved?
Discrepancies arise from limitations in early experimental methods (e.g., low-resolution NMR). Modern approaches include:
- Multisite natural-abundance NMR : Measures and KIEs at 0.1% precision, aligning with high-level DFT predictions (e.g., M06-2X/cc-pVTZ) .
- Tunneling corrections : One-dimensional Wentzel-Kramers-Brillouin (WKB) models account for quantum tunneling in transition states, reducing KIE prediction errors by 15–20% .
Q. What computational methods elucidate the transition-state topology of this compound rearrangements?
- ELF (Electron Localization Function) analysis : Identifies bond cleavage/formation regions (e.g., C–O bond rupture and C–C formation in Claisen rearrangement) .
- Energy profiles : DFT calculations (B3LYP/6-311+G(d,p)) reveal van der Waals complexes and π-interaction stabilization in Pd-catalyzed allylation pathways (ΔG‡ = 37.0 kJ/mol) .
- Nonthermal excitation studies : Femtosecond spectroscopy shows visible-light-induced rearrangement via electronic excited states, bypassing thermal activation .
Q. How do catalytic systems enhance regioselective C–H allylation using this compound?
- Ru(p-cymene)Cl catalysts : Promote ortho-allylation of aromatic carboxylates with this compound (yields >80%, allyl-to-vinyl ratio >15:1) via carboxylate-directed C–H activation .
- Palladium TPPTS complexes : Enable aqueous-phase allylation of phenol derivatives, with isomerization controlled by pH (optimal at pH 3–4) .
Q. What advanced kinetic methods improve efficiency in studying this compound reactions?
- Transient flow reactors : Automated systems coupled with inline NIR spectroscopy acquire 900 data points in 15 minutes, enabling rapid Arrhenius parameter estimation (E = 85–95 kJ/mol for Claisen rearrangement) .
- Excess acidity (X) analysis : Quantifies mechanistic shifts (A-1 vs. A-2 pathways) in acid-catalyzed hydrolysis using H acidity functions and deuterium isotope effects (k/k = 2.1–3.4) .
Q. Key Research Gaps and Recommendations
- Mechanistic ambiguity : Resolve conflicting A-1/A-2 pathway assignments in acid hydrolysis using ab initio molecular dynamics .
- Catalyst design : Explore chiral ligands for enantioselective allylations of heteroaromatics .
- Scalability : Optimize flow-reactor designs for gram-scale Claisen rearrangements with real-time impurity tracking .
Properties
IUPAC Name |
prop-2-enoxybenzene | |
---|---|---|
Source | PubChem | |
URL | https://pubchem.ncbi.nlm.nih.gov | |
Description | Data deposited in or computed by PubChem | |
InChI |
InChI=1S/C9H10O/c1-2-8-10-9-6-4-3-5-7-9/h2-7H,1,8H2 | |
Source | PubChem | |
URL | https://pubchem.ncbi.nlm.nih.gov | |
Description | Data deposited in or computed by PubChem | |
InChI Key |
POSICDHOUBKJKP-UHFFFAOYSA-N | |
Source | PubChem | |
URL | https://pubchem.ncbi.nlm.nih.gov | |
Description | Data deposited in or computed by PubChem | |
Canonical SMILES |
C=CCOC1=CC=CC=C1 | |
Source | PubChem | |
URL | https://pubchem.ncbi.nlm.nih.gov | |
Description | Data deposited in or computed by PubChem | |
Molecular Formula |
C9H10O | |
Source | PubChem | |
URL | https://pubchem.ncbi.nlm.nih.gov | |
Description | Data deposited in or computed by PubChem | |
DSSTOX Substance ID |
DTXSID6061943 | |
Record name | Allyl phenyl ether | |
Source | EPA DSSTox | |
URL | https://comptox.epa.gov/dashboard/DTXSID6061943 | |
Description | DSSTox provides a high quality public chemistry resource for supporting improved predictive toxicology. | |
Molecular Weight |
134.17 g/mol | |
Source | PubChem | |
URL | https://pubchem.ncbi.nlm.nih.gov | |
Description | Data deposited in or computed by PubChem | |
CAS No. |
1746-13-0 | |
Record name | Allyl phenyl ether | |
Source | CAS Common Chemistry | |
URL | https://commonchemistry.cas.org/detail?cas_rn=1746-13-0 | |
Description | CAS Common Chemistry is an open community resource for accessing chemical information. Nearly 500,000 chemical substances from CAS REGISTRY cover areas of community interest, including common and frequently regulated chemicals, and those relevant to high school and undergraduate chemistry classes. This chemical information, curated by our expert scientists, is provided in alignment with our mission as a division of the American Chemical Society. | |
Explanation | The data from CAS Common Chemistry is provided under a CC-BY-NC 4.0 license, unless otherwise stated. | |
Record name | Phenyl allyl ether | |
Source | ChemIDplus | |
URL | https://pubchem.ncbi.nlm.nih.gov/substance/?source=chemidplus&sourceid=0001746130 | |
Description | ChemIDplus is a free, web search system that provides access to the structure and nomenclature authority files used for the identification of chemical substances cited in National Library of Medicine (NLM) databases, including the TOXNET system. | |
Record name | Allyl phenyl ether | |
Source | DTP/NCI | |
URL | https://dtp.cancer.gov/dtpstandard/servlet/dwindex?searchtype=NSC&outputformat=html&searchlist=4746 | |
Description | The NCI Development Therapeutics Program (DTP) provides services and resources to the academic and private-sector research communities worldwide to facilitate the discovery and development of new cancer therapeutic agents. | |
Explanation | Unless otherwise indicated, all text within NCI products is free of copyright and may be reused without our permission. Credit the National Cancer Institute as the source. | |
Record name | Benzene, (2-propen-1-yloxy)- | |
Source | EPA Chemicals under the TSCA | |
URL | https://www.epa.gov/chemicals-under-tsca | |
Description | EPA Chemicals under the Toxic Substances Control Act (TSCA) collection contains information on chemicals and their regulations under TSCA, including non-confidential content from the TSCA Chemical Substance Inventory and Chemical Data Reporting. | |
Record name | Allyl phenyl ether | |
Source | EPA DSSTox | |
URL | https://comptox.epa.gov/dashboard/DTXSID6061943 | |
Description | DSSTox provides a high quality public chemistry resource for supporting improved predictive toxicology. | |
Record name | Allyl phenyl ether | |
Source | European Chemicals Agency (ECHA) | |
URL | https://echa.europa.eu/substance-information/-/substanceinfo/100.015.569 | |
Description | The European Chemicals Agency (ECHA) is an agency of the European Union which is the driving force among regulatory authorities in implementing the EU's groundbreaking chemicals legislation for the benefit of human health and the environment as well as for innovation and competitiveness. | |
Explanation | Use of the information, documents and data from the ECHA website is subject to the terms and conditions of this Legal Notice, and subject to other binding limitations provided for under applicable law, the information, documents and data made available on the ECHA website may be reproduced, distributed and/or used, totally or in part, for non-commercial purposes provided that ECHA is acknowledged as the source: "Source: European Chemicals Agency, http://echa.europa.eu/". Such acknowledgement must be included in each copy of the material. ECHA permits and encourages organisations and individuals to create links to the ECHA website under the following cumulative conditions: Links can only be made to webpages that provide a link to the Legal Notice page. | |
Record name | ALLYL PHENYL ETHER | |
Source | FDA Global Substance Registration System (GSRS) | |
URL | https://gsrs.ncats.nih.gov/ginas/app/beta/substances/26S07OSX4O | |
Description | The FDA Global Substance Registration System (GSRS) enables the efficient and accurate exchange of information on what substances are in regulated products. Instead of relying on names, which vary across regulatory domains, countries, and regions, the GSRS knowledge base makes it possible for substances to be defined by standardized, scientific descriptions. | |
Explanation | Unless otherwise noted, the contents of the FDA website (www.fda.gov), both text and graphics, are not copyrighted. They are in the public domain and may be republished, reprinted and otherwise used freely by anyone without the need to obtain permission from FDA. Credit to the U.S. Food and Drug Administration as the source is appreciated but not required. | |
Synthesis routes and methods I
Procedure details
Synthesis routes and methods II
Procedure details
Synthesis routes and methods III
Procedure details
Synthesis routes and methods IV
Procedure details
Synthesis routes and methods V
Procedure details
Retrosynthesis Analysis
AI-Powered Synthesis Planning: Our tool employs the Template_relevance Pistachio, Template_relevance Bkms_metabolic, Template_relevance Pistachio_ringbreaker, Template_relevance Reaxys, Template_relevance Reaxys_biocatalysis model, leveraging a vast database of chemical reactions to predict feasible synthetic routes.
One-Step Synthesis Focus: Specifically designed for one-step synthesis, it provides concise and direct routes for your target compounds, streamlining the synthesis process.
Accurate Predictions: Utilizing the extensive PISTACHIO, BKMS_METABOLIC, PISTACHIO_RINGBREAKER, REAXYS, REAXYS_BIOCATALYSIS database, our tool offers high-accuracy predictions, reflecting the latest in chemical research and data.
Strategy Settings
Precursor scoring | Relevance Heuristic |
---|---|
Min. plausibility | 0.01 |
Model | Template_relevance |
Template Set | Pistachio/Bkms_metabolic/Pistachio_ringbreaker/Reaxys/Reaxys_biocatalysis |
Top-N result to add to graph | 6 |
Feasible Synthetic Routes
Disclaimer and Information on In-Vitro Research Products
Please be aware that all articles and product information presented on BenchChem are intended solely for informational purposes. The products available for purchase on BenchChem are specifically designed for in-vitro studies, which are conducted outside of living organisms. In-vitro studies, derived from the Latin term "in glass," involve experiments performed in controlled laboratory settings using cells or tissues. It is important to note that these products are not categorized as medicines or drugs, and they have not received approval from the FDA for the prevention, treatment, or cure of any medical condition, ailment, or disease. We must emphasize that any form of bodily introduction of these products into humans or animals is strictly prohibited by law. It is essential to adhere to these guidelines to ensure compliance with legal and ethical standards in research and experimentation.