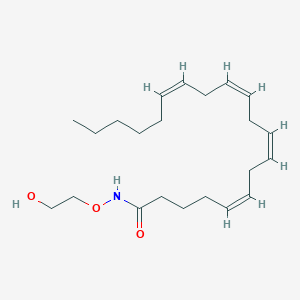
N-arachidonoyl-O-(2-hydroxyethyl)hydroxylamine
Overview
Description
Oxy-Arachidonoyl Ethanolamide: is a bioactive lipid mediator that belongs to the family of fatty acid ethanolamides. It is a derivative of arachidonic acid and ethanolamine, and it is known for its role in various physiological processes. This compound is also referred to as anandamide, which is derived from the Sanskrit word “ananda,” meaning bliss or delight. Oxy-Arachidonoyl Ethanolamide is an endogenous cannabinoid neurotransmitter that binds to cannabinoid receptors in the body, influencing pain, mood, appetite, and memory .
Mechanism of Action
Target of Action
The primary target of N-arachidonoyl-O-(2-hydroxyethyl)hydroxylamine is the Cannabinoid receptor 1 (CB1) . This receptor mediates many cannabinoid-induced effects, including effects on food intake, memory loss, gastrointestinal motility, catalepsy, ambulatory activity, anxiety, and chronic pain .
Mode of Action
The compound interacts with its target, the CB1 receptor, and induces a series of changes. The signaling typically involves a reduction in cyclic AMP . At high doses, the signal transduction involves G-protein alpha-i protein activation and subsequent inhibition of mitochondrial soluble adenylate cyclase, leading to a decrease in cyclic AMP concentration .
Biochemical Pathways
The compound affects several biochemical pathways. In the hypothalamus, it may have a dual effect on mitochondrial respiration depending on the agonist dose . It also inhibits leptin-induced reactive oxygen species (ROS) formation and mediates cannabinoid-induced increase in SREBF1 and FASN gene expression . In the hippocampus, it regulates cellular respiration and energy production in response to cannabinoids .
Pharmacokinetics
It is known to be a small molecular drug , which suggests that it may have good bioavailability and can be distributed throughout the body effectively.
Result of Action
The compound’s action results in a variety of molecular and cellular effects. It drives the release of orexigenic beta-endorphin from hypothalamic POMC neurons, promoting food intake . It also reduces excitatory synaptic transmission and inhibits voltage-gated Ca(2+) channels in a constitutive, as well as agonist-dependent manner .
Action Environment
Environmental factors can influence the compound’s action, efficacy, and stability. For instance, in the context of inflammation, this compound and similar N-acylethanolamines may play a role and activate ion channels, revealing potential applications in inflammatory diseases and neuropharmacology.
Biochemical Analysis
Biochemical Properties
N-arachidonoyl-O-(2-hydroxyethyl)hydroxylamine has been found to interact with various enzymes and proteins. It has a greater affinity for the central cannabinoid (CB1) receptor with Ki values of 0.07 and 0.18 µM for hCB1 and hCB2, respectively . This compound is the first known fatty acid amide with a reversed CB1/CB2 affinity ratio .
Cellular Effects
This compound has been shown to have various effects on cells. It mediates many cannabinoid-induced effects, acting on food intake, memory loss, gastrointestinal motility, catalepsy, ambulatory activity, anxiety, and chronic pain . It also influences cell function, including impacts on cell signaling pathways, gene expression, and cellular metabolism .
Molecular Mechanism
At the molecular level, this compound exerts its effects through binding interactions with biomolecules, enzyme inhibition or activation, and changes in gene expression . It typically involves reduction in cyclic AMP .
Temporal Effects in Laboratory Settings
The effects of this compound over time in laboratory settings are yet to be fully understood. It has been shown to have agonist activity on human recombinant TRPV1-mediated enhancement of intracellular calcium concentration in HEK293 cells .
Metabolic Pathways
The metabolic pathways that this compound is involved in are not fully understood. It is known to interact with the cannabinoid receptor 1 (CB1) .
Transport and Distribution
The transport and distribution of this compound within cells and tissues are not fully understood. It is known to interact with the cannabinoid receptor 1 (CB1) .
Subcellular Localization
The subcellular localization of this compound and its effects on activity or function are not fully understood. It is known to interact with the cannabinoid receptor 1 (CB1) .
Preparation Methods
Synthetic Routes and Reaction Conditions: The synthesis of oxy-Arachidonoyl Ethanolamide typically involves the reaction of arachidonic acid with ethanolamine. One common method is enzymatic synthesis using lipase as a catalyst. The process begins with the extraction of free fatty acids from arachidonic acid-rich oil. The arachidonic acid is then enriched through urea inclusion and silver nitrate solution fractionation. The enriched arachidonic acid is reacted with ethanolamine in hexane, using Novozym 435 lipase as a catalyst. This results in the formation of oxy-Arachidonoyl Ethanolamide with high yield and purity .
Industrial Production Methods: Industrial production of oxy-Arachidonoyl Ethanolamide follows similar synthetic routes but on a larger scale. The process involves optimizing reaction conditions to achieve high yields and purity. The use of native oils as acyl donors and mixed solvents as reaction media can enhance the efficiency of the synthesis. The product is then purified through crystallization processes to obtain highly pure oxy-Arachidonoyl Ethanolamide .
Chemical Reactions Analysis
Types of Reactions: Oxy-Arachidonoyl Ethanolamide undergoes various chemical reactions, including:
Oxidation: This reaction involves the addition of oxygen to the compound, leading to the formation of oxidized derivatives.
Reduction: This reaction involves the removal of oxygen or the addition of hydrogen, resulting in reduced forms of the compound.
Substitution: This reaction involves the replacement of one functional group with another, leading to the formation of substituted derivatives.
Common Reagents and Conditions:
Oxidation: Common reagents include hydrogen peroxide and oxygen. The reaction is typically carried out under mild conditions.
Reduction: Common reagents include sodium borohydride and hydrogen gas. The reaction is usually carried out under controlled conditions to prevent over-reduction.
Substitution: Common reagents include halogens and nucleophiles. The reaction conditions vary depending on the specific substitution being carried out.
Major Products Formed:
Oxidation: Oxidized derivatives of oxy-Arachidonoyl Ethanolamide.
Reduction: Reduced forms of oxy-Arachidonoyl Ethanolamide.
Substitution: Substituted derivatives with various functional groups
Scientific Research Applications
Oxy-Arachidonoyl Ethanolamide has a wide range of scientific research applications, including:
Chemistry: It is used as a model compound to study the behavior of fatty acid ethanolamides and their interactions with other molecules.
Biology: It is studied for its role in cellular signaling and its effects on various physiological processes, such as pain modulation, appetite regulation, and mood enhancement.
Medicine: It has potential therapeutic applications in the treatment of pain, inflammation, anxiety, and neurodegenerative diseases. Its ability to bind to cannabinoid receptors makes it a promising candidate for drug development.
Industry: It is used in the production of lubricants, surfactants, and cosmetics due to its unique bioactive properties .
Comparison with Similar Compounds
Oxy-Arachidonoyl Ethanolamide is unique among fatty acid ethanolamides due to its specific structure and bioactivity. Similar compounds include:
Oleoyl Ethanolamide: Known for its role in appetite regulation and lipid metabolism.
Palmitoyl Ethanolamide: Known for its anti-inflammatory and neuroprotective effects.
Stearoyl Ethanolamide: Known for its anti-inflammatory properties and potential therapeutic applications in pain management.
These compounds share similar structural features but differ in their specific fatty acid chains and bioactivities. Oxy-Arachidonoyl Ethanolamide stands out due to its strong affinity for cannabinoid receptors and its wide range of physiological effects .
Properties
IUPAC Name |
(5Z,8Z,11Z,14Z)-N-(2-hydroxyethoxy)icosa-5,8,11,14-tetraenamide | |
---|---|---|
Source | PubChem | |
URL | https://pubchem.ncbi.nlm.nih.gov | |
Description | Data deposited in or computed by PubChem | |
InChI |
InChI=1S/C22H37NO3/c1-2-3-4-5-6-7-8-9-10-11-12-13-14-15-16-17-18-19-22(25)23-26-21-20-24/h6-7,9-10,12-13,15-16,24H,2-5,8,11,14,17-21H2,1H3,(H,23,25)/b7-6-,10-9-,13-12-,16-15- | |
Source | PubChem | |
URL | https://pubchem.ncbi.nlm.nih.gov | |
Description | Data deposited in or computed by PubChem | |
InChI Key |
ANMKTCMIIFBOGG-DOFZRALJSA-N | |
Source | PubChem | |
URL | https://pubchem.ncbi.nlm.nih.gov | |
Description | Data deposited in or computed by PubChem | |
Canonical SMILES |
CCCCCC=CCC=CCC=CCC=CCCCC(=O)NOCCO | |
Source | PubChem | |
URL | https://pubchem.ncbi.nlm.nih.gov | |
Description | Data deposited in or computed by PubChem | |
Isomeric SMILES |
CCCCC/C=C\C/C=C\C/C=C\C/C=C\CCCC(=O)NOCCO | |
Source | PubChem | |
URL | https://pubchem.ncbi.nlm.nih.gov | |
Description | Data deposited in or computed by PubChem | |
Molecular Formula |
C22H37NO3 | |
Source | PubChem | |
URL | https://pubchem.ncbi.nlm.nih.gov | |
Description | Data deposited in or computed by PubChem | |
Molecular Weight |
363.5 g/mol | |
Source | PubChem | |
URL | https://pubchem.ncbi.nlm.nih.gov | |
Description | Data deposited in or computed by PubChem | |
Retrosynthesis Analysis
AI-Powered Synthesis Planning: Our tool employs the Template_relevance Pistachio, Template_relevance Bkms_metabolic, Template_relevance Pistachio_ringbreaker, Template_relevance Reaxys, Template_relevance Reaxys_biocatalysis model, leveraging a vast database of chemical reactions to predict feasible synthetic routes.
One-Step Synthesis Focus: Specifically designed for one-step synthesis, it provides concise and direct routes for your target compounds, streamlining the synthesis process.
Accurate Predictions: Utilizing the extensive PISTACHIO, BKMS_METABOLIC, PISTACHIO_RINGBREAKER, REAXYS, REAXYS_BIOCATALYSIS database, our tool offers high-accuracy predictions, reflecting the latest in chemical research and data.
Strategy Settings
Precursor scoring | Relevance Heuristic |
---|---|
Min. plausibility | 0.01 |
Model | Template_relevance |
Template Set | Pistachio/Bkms_metabolic/Pistachio_ringbreaker/Reaxys/Reaxys_biocatalysis |
Top-N result to add to graph | 6 |
Feasible Synthetic Routes
Disclaimer and Information on In-Vitro Research Products
Please be aware that all articles and product information presented on BenchChem are intended solely for informational purposes. The products available for purchase on BenchChem are specifically designed for in-vitro studies, which are conducted outside of living organisms. In-vitro studies, derived from the Latin term "in glass," involve experiments performed in controlled laboratory settings using cells or tissues. It is important to note that these products are not categorized as medicines or drugs, and they have not received approval from the FDA for the prevention, treatment, or cure of any medical condition, ailment, or disease. We must emphasize that any form of bodily introduction of these products into humans or animals is strictly prohibited by law. It is essential to adhere to these guidelines to ensure compliance with legal and ethical standards in research and experimentation.