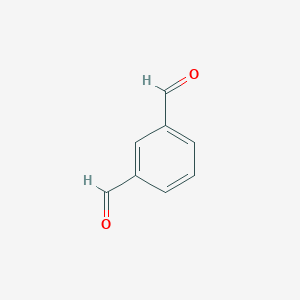
Isophthalaldehyde
Overview
Description
Isophthalaldehyde, also known as 1,3-benzenedicarboxaldehyde, is an organic compound with the molecular formula C8H6O2. It is one of the three isomers of benzene dicarbaldehyde, a reduced analog of phthalic acid. This compound appears as a colorless solid, although commercial samples often have a yellowish tint . This compound is primarily used in the synthesis of polymers and coordination complexes .
Mechanism of Action
. .
Mode of Action
Isophthalaldehyde is bifunctional, meaning it has two formyl groups . This allows it to interact with its targets (di- or triamines) in a unique way. It forms a variety of Schiff base derivatives through these interactions . These derivatives are then used in the formation of polymers or covalent organic frameworks .
Biochemical Pathways
It is known that this compound can form polymers or covalent organic frameworks upon reaction with di- or triamines . These frameworks can have various applications, including use in metal coordination complexes .
Pharmacokinetics
Its chemical properties, such as its molecular weight of 134134 g/mol and its density of 1.395 g/cm^3 , can influence its bioavailability.
Result of Action
The result of this compound’s action is the formation of polymers or covalent organic frameworks . These frameworks can be used in various applications, including the creation of metal coordination complexes .
Action Environment
The action of this compound can be influenced by various environmental factors. For example, its solid state and color (it is a colorless solid, although commercial samples often appear yellowish ) can affect its stability. Furthermore, its melting point of 89.5 °C can influence its efficacy under different temperature conditions.
Biochemical Analysis
. . .
Biochemical Properties
Isophthalaldehyde, being bifunctional (having two formyl groups), allows the formation of polymers or covalent organic frameworks upon reaction with di- or triamines . They also find use in metal coordination complexes .
Molecular Mechanism
It is known to form a variety of Schiff base derivatives , which could potentially interact with various biomolecules
Preparation Methods
Synthetic Routes and Reaction Conditions: Isophthalaldehyde can be synthesized through several methods:
Sommelet Reaction: This involves the reaction of α,α’-diamino-ortho-xylene with hexamethylenetetramine in the presence of hydrochloric acid and acetic acid.
Catalytic Oxidation: m-Xylene is used as the raw material for catalytic oxidation to obtain this compound.
Reduction of Isophthaloyl Chloride: This method involves the reduction of isophthaloyl chloride using lithium tri-t-butoxyaluminumhydride.
Industrial Production Methods: The industrial production of this compound typically involves the catalytic oxidation of m-xylene, which offers high yield and purity while minimizing waste and pollution .
Chemical Reactions Analysis
Isophthalaldehyde undergoes various chemical reactions, including:
Oxidation: It can be oxidized to form isophthalic acid.
Reduction: Reduction of this compound can yield isophthalyl alcohol.
Substitution: It participates in base-catalyzed Knoevenagel condensation reactions.
Schiff Base Formation: this compound reacts with amines to form Schiff bases, which are useful in the synthesis of polymers and coordination complexes.
Common Reagents and Conditions:
Oxidation: Common oxidizing agents include potassium permanganate and chromium trioxide.
Reduction: Reducing agents such as sodium borohydride and lithium aluminum hydride are used.
Knoevenagel Condensation: This reaction typically requires a base catalyst like piperidine or pyridine.
Major Products:
Isophthalic Acid: Formed through oxidation.
Isophthalyl Alcohol: Formed through reduction.
Schiff Bases: Formed through reactions with amines.
Scientific Research Applications
Isophthalaldehyde has diverse applications in scientific research:
Biology: Schiff bases derived from this compound are studied for their potential biological activities.
Medicine: Research is ongoing into the use of this compound derivatives in drug development.
Comparison with Similar Compounds
Isophthalaldehyde is one of three isomers of benzene dicarbaldehyde. The other two isomers are:
Phthalaldehyde (1,2-benzenedicarboxaldehyde): Used in the synthesis of pharmaceuticals and as a disinfectant.
Terephthalaldehyde (1,4-benzenedicarboxaldehyde): Used in the production of polymers and as a precursor for various chemical syntheses.
Uniqueness: this compound’s unique position of the formyl groups (1,3-positions) allows for the formation of specific Schiff bases and coordination complexes that are not possible with the other isomers. This makes it particularly valuable in the synthesis of specialized polymers and materials .
Properties
IUPAC Name |
benzene-1,3-dicarbaldehyde | |
---|---|---|
Source | PubChem | |
URL | https://pubchem.ncbi.nlm.nih.gov | |
Description | Data deposited in or computed by PubChem | |
InChI |
InChI=1S/C8H6O2/c9-5-7-2-1-3-8(4-7)6-10/h1-6H | |
Source | PubChem | |
URL | https://pubchem.ncbi.nlm.nih.gov | |
Description | Data deposited in or computed by PubChem | |
InChI Key |
IZALUMVGBVKPJD-UHFFFAOYSA-N | |
Source | PubChem | |
URL | https://pubchem.ncbi.nlm.nih.gov | |
Description | Data deposited in or computed by PubChem | |
Canonical SMILES |
C1=CC(=CC(=C1)C=O)C=O | |
Source | PubChem | |
URL | https://pubchem.ncbi.nlm.nih.gov | |
Description | Data deposited in or computed by PubChem | |
Molecular Formula |
C8H6O2 | |
Source | PubChem | |
URL | https://pubchem.ncbi.nlm.nih.gov | |
Description | Data deposited in or computed by PubChem | |
DSSTOX Substance ID |
DTXSID30870718 | |
Record name | 1,3-Benzenedicarboxaldehyde | |
Source | EPA DSSTox | |
URL | https://comptox.epa.gov/dashboard/DTXSID30870718 | |
Description | DSSTox provides a high quality public chemistry resource for supporting improved predictive toxicology. | |
Molecular Weight |
134.13 g/mol | |
Source | PubChem | |
URL | https://pubchem.ncbi.nlm.nih.gov | |
Description | Data deposited in or computed by PubChem | |
Boiling Point |
246 °C | |
Record name | Isophthalaldehyde | |
Source | Hazardous Substances Data Bank (HSDB) | |
URL | https://pubchem.ncbi.nlm.nih.gov/source/hsdb/8459 | |
Description | The Hazardous Substances Data Bank (HSDB) is a toxicology database that focuses on the toxicology of potentially hazardous chemicals. It provides information on human exposure, industrial hygiene, emergency handling procedures, environmental fate, regulatory requirements, nanomaterials, and related areas. The information in HSDB has been assessed by a Scientific Review Panel. | |
Solubility |
Slightly soluble in water, Slightly soluble in ethyl ether, chloroform; very soluble in ethanol; soluble in acetone, benzene | |
Record name | Isophthalaldehyde | |
Source | Hazardous Substances Data Bank (HSDB) | |
URL | https://pubchem.ncbi.nlm.nih.gov/source/hsdb/8459 | |
Description | The Hazardous Substances Data Bank (HSDB) is a toxicology database that focuses on the toxicology of potentially hazardous chemicals. It provides information on human exposure, industrial hygiene, emergency handling procedures, environmental fate, regulatory requirements, nanomaterials, and related areas. The information in HSDB has been assessed by a Scientific Review Panel. | |
Color/Form |
Needles from dilute alcohol | |
CAS No. |
626-19-7, 30025-33-3 | |
Record name | 1,3-Benzenedicarboxaldehyde | |
Source | CAS Common Chemistry | |
URL | https://commonchemistry.cas.org/detail?cas_rn=626-19-7 | |
Description | CAS Common Chemistry is an open community resource for accessing chemical information. Nearly 500,000 chemical substances from CAS REGISTRY cover areas of community interest, including common and frequently regulated chemicals, and those relevant to high school and undergraduate chemistry classes. This chemical information, curated by our expert scientists, is provided in alignment with our mission as a division of the American Chemical Society. | |
Explanation | The data from CAS Common Chemistry is provided under a CC-BY-NC 4.0 license, unless otherwise stated. | |
Record name | Isophthalaldehyde | |
Source | ChemIDplus | |
URL | https://pubchem.ncbi.nlm.nih.gov/substance/?source=chemidplus&sourceid=0000626197 | |
Description | ChemIDplus is a free, web search system that provides access to the structure and nomenclature authority files used for the identification of chemical substances cited in National Library of Medicine (NLM) databases, including the TOXNET system. | |
Record name | Benzenedicarboxaldehyde | |
Source | ChemIDplus | |
URL | https://pubchem.ncbi.nlm.nih.gov/substance/?source=chemidplus&sourceid=0030025333 | |
Description | ChemIDplus is a free, web search system that provides access to the structure and nomenclature authority files used for the identification of chemical substances cited in National Library of Medicine (NLM) databases, including the TOXNET system. | |
Record name | Isophthalaldehyde | |
Source | DTP/NCI | |
URL | https://dtp.cancer.gov/dtpstandard/servlet/dwindex?searchtype=NSC&outputformat=html&searchlist=5092 | |
Description | The NCI Development Therapeutics Program (DTP) provides services and resources to the academic and private-sector research communities worldwide to facilitate the discovery and development of new cancer therapeutic agents. | |
Explanation | Unless otherwise indicated, all text within NCI products is free of copyright and may be reused without our permission. Credit the National Cancer Institute as the source. | |
Record name | 1,3-Benzenedicarboxaldehyde | |
Source | EPA DSSTox | |
URL | https://comptox.epa.gov/dashboard/DTXSID30870718 | |
Description | DSSTox provides a high quality public chemistry resource for supporting improved predictive toxicology. | |
Record name | Isophthalaldehyde | |
Source | European Chemicals Agency (ECHA) | |
URL | https://echa.europa.eu/substance-information/-/substanceinfo/100.009.942 | |
Description | The European Chemicals Agency (ECHA) is an agency of the European Union which is the driving force among regulatory authorities in implementing the EU's groundbreaking chemicals legislation for the benefit of human health and the environment as well as for innovation and competitiveness. | |
Explanation | Use of the information, documents and data from the ECHA website is subject to the terms and conditions of this Legal Notice, and subject to other binding limitations provided for under applicable law, the information, documents and data made available on the ECHA website may be reproduced, distributed and/or used, totally or in part, for non-commercial purposes provided that ECHA is acknowledged as the source: "Source: European Chemicals Agency, http://echa.europa.eu/". Such acknowledgement must be included in each copy of the material. ECHA permits and encourages organisations and individuals to create links to the ECHA website under the following cumulative conditions: Links can only be made to webpages that provide a link to the Legal Notice page. | |
Record name | ISO-PHTHALALDEHYDE | |
Source | FDA Global Substance Registration System (GSRS) | |
URL | https://gsrs.ncats.nih.gov/ginas/app/beta/substances/LU162B2N9X | |
Description | The FDA Global Substance Registration System (GSRS) enables the efficient and accurate exchange of information on what substances are in regulated products. Instead of relying on names, which vary across regulatory domains, countries, and regions, the GSRS knowledge base makes it possible for substances to be defined by standardized, scientific descriptions. | |
Explanation | Unless otherwise noted, the contents of the FDA website (www.fda.gov), both text and graphics, are not copyrighted. They are in the public domain and may be republished, reprinted and otherwise used freely by anyone without the need to obtain permission from FDA. Credit to the U.S. Food and Drug Administration as the source is appreciated but not required. | |
Record name | Isophthalaldehyde | |
Source | Hazardous Substances Data Bank (HSDB) | |
URL | https://pubchem.ncbi.nlm.nih.gov/source/hsdb/8459 | |
Description | The Hazardous Substances Data Bank (HSDB) is a toxicology database that focuses on the toxicology of potentially hazardous chemicals. It provides information on human exposure, industrial hygiene, emergency handling procedures, environmental fate, regulatory requirements, nanomaterials, and related areas. The information in HSDB has been assessed by a Scientific Review Panel. | |
Melting Point |
89 °C | |
Record name | Isophthalaldehyde | |
Source | Hazardous Substances Data Bank (HSDB) | |
URL | https://pubchem.ncbi.nlm.nih.gov/source/hsdb/8459 | |
Description | The Hazardous Substances Data Bank (HSDB) is a toxicology database that focuses on the toxicology of potentially hazardous chemicals. It provides information on human exposure, industrial hygiene, emergency handling procedures, environmental fate, regulatory requirements, nanomaterials, and related areas. The information in HSDB has been assessed by a Scientific Review Panel. | |
Retrosynthesis Analysis
AI-Powered Synthesis Planning: Our tool employs the Template_relevance Pistachio, Template_relevance Bkms_metabolic, Template_relevance Pistachio_ringbreaker, Template_relevance Reaxys, Template_relevance Reaxys_biocatalysis model, leveraging a vast database of chemical reactions to predict feasible synthetic routes.
One-Step Synthesis Focus: Specifically designed for one-step synthesis, it provides concise and direct routes for your target compounds, streamlining the synthesis process.
Accurate Predictions: Utilizing the extensive PISTACHIO, BKMS_METABOLIC, PISTACHIO_RINGBREAKER, REAXYS, REAXYS_BIOCATALYSIS database, our tool offers high-accuracy predictions, reflecting the latest in chemical research and data.
Strategy Settings
Precursor scoring | Relevance Heuristic |
---|---|
Min. plausibility | 0.01 |
Model | Template_relevance |
Template Set | Pistachio/Bkms_metabolic/Pistachio_ringbreaker/Reaxys/Reaxys_biocatalysis |
Top-N result to add to graph | 6 |
Feasible Synthetic Routes
Q1: What is the molecular formula and weight of isophthalaldehyde?
A1: this compound is represented by the molecular formula C8H6O2 and has a molecular weight of 134.13 g/mol.
Q2: What spectroscopic techniques are commonly employed to characterize this compound?
A2: Researchers utilize a combination of spectroscopic methods for comprehensive characterization, including Infrared (IR) spectroscopy, Ultraviolet-Visible (UV-Vis) spectroscopy, Nuclear Magnetic Resonance (NMR) spectroscopy (both 1H and 13C), and Mass Spectrometry (MS). [, , , , , , ]
Q3: What solvents are commonly used for reactions involving this compound?
A3: this compound exhibits solubility in a range of organic solvents, including methanol, ethanol, dichloromethane, and N,N-dimethylacetamide, facilitating its use in diverse synthetic transformations. [, , , , ]
Q4: How does the stability of this compound in solution vary?
A4: While generally stable in common organic solvents, this compound's stability in aqueous solutions can be influenced by pH. Under strongly alkaline conditions, it undergoes a reversible reaction with hydroxide ions to form a geminal diol anion. []
Q5: Can this compound be polymerized?
A5: Yes, this compound can be polymerized through various methods. For example, it undergoes a Tishchenko reaction in the presence of ethylmagnesium bromide-(−)-sparteine complex and aluminum alkoxides to yield polyesters. []
Q6: What role does this compound play in the synthesis of covalent organic frameworks (COFs)?
A6: this compound serves as a key building block in the construction of COFs. Its condensation with linear hydrazine linkers, under solvothermal or microwave heating conditions, affords porous 2D COFs with distinct pore structures. []
Q7: How is computational chemistry employed in research on this compound and its derivatives?
A7: Computational techniques, such as Density Functional Theory (DFT) calculations and molecular mechanics simulations, provide valuable insights into the structural, electronic, and energetic properties of this compound-derived compounds. These methods aid in understanding reaction mechanisms, predicting spectroscopic data, and designing novel materials. [, , ]
Q8: How do structural modifications of this compound impact the properties of its derivatives?
A8: Introducing substituents onto the aromatic ring of this compound significantly influences the reactivity and properties of the resulting compounds. For instance, incorporating electron-donating or electron-withdrawing groups alters the electronic distribution, impacting their coordination chemistry and biological activity. [, , ]
Q9: What analytical techniques are used to monitor the reactions involving this compound?
A9: Researchers employ a combination of techniques to monitor reactions involving this compound, including thin-layer chromatography (TLC), gas chromatography (GC), and high-performance liquid chromatography (HPLC). [, , ]
Q10: How is the purity of this compound and its derivatives assessed?
A10: Purity assessment of this compound and its derivatives commonly involves techniques like melting point determination, elemental analysis, and spectroscopic methods such as NMR and MS. [, , ]
Disclaimer and Information on In-Vitro Research Products
Please be aware that all articles and product information presented on BenchChem are intended solely for informational purposes. The products available for purchase on BenchChem are specifically designed for in-vitro studies, which are conducted outside of living organisms. In-vitro studies, derived from the Latin term "in glass," involve experiments performed in controlled laboratory settings using cells or tissues. It is important to note that these products are not categorized as medicines or drugs, and they have not received approval from the FDA for the prevention, treatment, or cure of any medical condition, ailment, or disease. We must emphasize that any form of bodily introduction of these products into humans or animals is strictly prohibited by law. It is essential to adhere to these guidelines to ensure compliance with legal and ethical standards in research and experimentation.