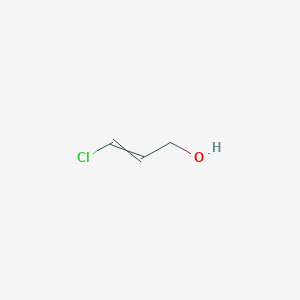
3-Chloroallyl alcohol
Overview
Description
Mechanism of Action
Target of Action
The primary target of 3-Chloroallyl alcohol (3-CAA) is hepatic alcohol dehydrogenases . These enzymes play a crucial role in the metabolism of alcohols, including 3-CAA .
Mode of Action
3-CAA, like ethanol, is a primary alcohol and is metabolized largely by hepatic alcohol dehydrogenases . The interaction of 3-CAA with these enzymes leads to its metabolism, producing various metabolites .
Biochemical Pathways
The metabolism of 3-CAA by hepatic alcohol dehydrogenases is part of the broader alcohol metabolic pathway . This pathway is crucial in the body’s processing of alcohol-based compounds, leading to their breakdown and eventual elimination .
Pharmacokinetics
A physiologically based pharmacokinetic (PBPK) model for 3-CAA has been developed . This model predicts that oral gavage results in a 6-fold higher maximum blood concentration (Cmax) than the same dose administered in drinking water, but in similar area under the curve (AUC) values . This suggests that the route of administration can significantly impact the bioavailability of 3-CAA .
Result of Action
The metabolism of 3-CAA by hepatic alcohol dehydrogenases leads to the production of a reactive metabolite . This metabolite is associated with severe toxicity, such as lethality, from 3-CAA . It’s important to note that the specific molecular and cellular effects of 3-caa’s action may vary depending on several factors, including the dose and route of administration .
Action Environment
Environmental factors can influence the action, efficacy, and stability of 3-CAA. For instance, 3-CAA can be found in the environment following the application of certain plant protection products . It is also formed in groundwater following the injection of 1,3-dichloropropene, a fumigant used to control nematodes . These environmental occurrences could potentially affect the exposure levels and subsequent action of 3-CAA .
Biochemical Analysis
Biochemical Properties
3-Chloroallyl alcohol, like ethanol, is a primary alcohol and is metabolized largely by hepatic alcohol dehydrogenases . This interaction with enzymes plays a significant role in its biochemical reactions. The nature of these interactions involves the conversion of this compound into other compounds through metabolic processes .
Cellular Effects
The effects of this compound on cells and cellular processes are significant. It influences cell function, including impacts on cell signaling pathways, gene expression, and cellular metabolism . The exact mechanisms of these influences are complex and involve a variety of biochemical reactions and interactions with enzymes .
Molecular Mechanism
At the molecular level, this compound exerts its effects through binding interactions with biomolecules, enzyme inhibition or activation, and changes in gene expression . The compound’s interactions with hepatic alcohol dehydrogenases, for example, play a crucial role in its metabolism .
Temporal Effects in Laboratory Settings
In laboratory settings, the effects of this compound can change over time. This includes information on the product’s stability, degradation, and any long-term effects on cellular function observed in in vitro or in vivo studies .
Dosage Effects in Animal Models
The effects of this compound vary with different dosages in animal models. At the same administered dose, oral gavage produces a 6-fold higher maximum blood concentration than drinking water, but a similar area under the curve, and that maximum blood concentration was more strongly correlated with severe toxicity and lethality .
Metabolic Pathways
This compound is involved in several metabolic pathways. It interacts with enzymes such as hepatic alcohol dehydrogenases, which play a significant role in its metabolism .
Transport and Distribution
The transport and distribution of this compound within cells and tissues involve various processes. These could include interactions with transporters or binding proteins, as well as effects on its localization or accumulation .
Subcellular Localization
The subcellular localization of this compound and its effects on activity or function are complex. This could include any targeting signals or post-translational modifications that direct it to specific compartments or organelles .
Preparation Methods
Synthetic Routes and Reaction Conditions: 3-Chloroallyl alcohol can be synthesized through the reaction of trichloropropane with sodium acetate in the presence of dimethylformamide and a catalyst such as dibenzo-18-crown-6. The reaction is carried out at temperatures ranging from 115°C to 140°C. The product, 3-acetoxy-2-chloro-1-propene, is then hydrolyzed to yield this compound .
Industrial Production Methods: Industrial production methods for this compound typically involve the same synthetic route but on a larger scale. The use of continuous flow reactors and optimized reaction conditions ensures high yield and purity of the final product.
Types of Reactions:
Oxidation: this compound can undergo oxidation reactions to form corresponding aldehydes or carboxylic acids.
Reduction: Reduction of this compound can lead to the formation of 3-chloropropanol.
Substitution: The chlorine atom in this compound can be substituted by other nucleophiles, such as hydroxide ions, to form different derivatives.
Common Reagents and Conditions:
Oxidation: Common oxidizing agents include potassium permanganate and chromium trioxide.
Reduction: Reducing agents such as lithium aluminum hydride or sodium borohydride are used.
Substitution: Nucleophilic substitution reactions often involve reagents like sodium hydroxide or potassium hydroxide.
Major Products Formed:
Oxidation: 3-Chloroacrolein or 3-chloropropionic acid.
Reduction: 3-Chloropropanol.
Substitution: Various substituted allyl alcohols depending on the nucleophile used.
Scientific Research Applications
3-Chloroallyl alcohol has several applications in scientific research:
Chemistry: It is used as an intermediate in the synthesis of other organic compounds, including pharmaceuticals and agrochemicals.
Biology: Studies have explored its potential genotoxic effects and its metabolism in living organisms.
Medicine: Research is ongoing to understand its potential therapeutic applications and toxicological profile.
Industry: It is used in the production of polymers, resins, and other industrial chemicals.
Comparison with Similar Compounds
2-Chloroallyl alcohol: Similar in structure but with the chlorine atom attached to a different carbon.
3-Chloropropanol: A reduction product of 3-chloroallyl alcohol.
3-Chloroacrolein: An oxidation product of this compound.
Uniqueness: this compound is unique due to its specific reactivity and the position of the chlorine atom, which influences its chemical behavior and potential applications. Its ability to undergo various chemical reactions makes it a versatile compound in synthetic chemistry.
Properties
IUPAC Name |
3-chloroprop-2-en-1-ol | |
---|---|---|
Source | PubChem | |
URL | https://pubchem.ncbi.nlm.nih.gov | |
Description | Data deposited in or computed by PubChem | |
InChI |
InChI=1S/C3H5ClO/c4-2-1-3-5/h1-2,5H,3H2 | |
Source | PubChem | |
URL | https://pubchem.ncbi.nlm.nih.gov | |
Description | Data deposited in or computed by PubChem | |
InChI Key |
HJGHXDNIPAWLLE-UHFFFAOYSA-N | |
Source | PubChem | |
URL | https://pubchem.ncbi.nlm.nih.gov | |
Description | Data deposited in or computed by PubChem | |
Canonical SMILES |
C(C=CCl)O | |
Source | PubChem | |
URL | https://pubchem.ncbi.nlm.nih.gov | |
Description | Data deposited in or computed by PubChem | |
Molecular Formula |
C3H5ClO | |
Source | PubChem | |
URL | https://pubchem.ncbi.nlm.nih.gov | |
Description | Data deposited in or computed by PubChem | |
DSSTOX Substance ID |
DTXSID5041481 | |
Record name | 3-Chloroallyl alcohol | |
Source | EPA DSSTox | |
URL | https://comptox.epa.gov/dashboard/DTXSID5041481 | |
Description | DSSTox provides a high quality public chemistry resource for supporting improved predictive toxicology. | |
Molecular Weight |
92.52 g/mol | |
Source | PubChem | |
URL | https://pubchem.ncbi.nlm.nih.gov | |
Description | Data deposited in or computed by PubChem | |
CAS No. |
29560-84-7 | |
Record name | 2-Propen-1-ol, 3-chloro- | |
Source | CAS Common Chemistry | |
URL | https://commonchemistry.cas.org/detail?cas_rn=29560-84-7 | |
Description | CAS Common Chemistry is an open community resource for accessing chemical information. Nearly 500,000 chemical substances from CAS REGISTRY cover areas of community interest, including common and frequently regulated chemicals, and those relevant to high school and undergraduate chemistry classes. This chemical information, curated by our expert scientists, is provided in alignment with our mission as a division of the American Chemical Society. | |
Explanation | The data from CAS Common Chemistry is provided under a CC-BY-NC 4.0 license, unless otherwise stated. | |
Record name | 3-Chloroallyl alcohol | |
Source | ChemIDplus | |
URL | https://pubchem.ncbi.nlm.nih.gov/substance/?source=chemidplus&sourceid=0029560847 | |
Description | ChemIDplus is a free, web search system that provides access to the structure and nomenclature authority files used for the identification of chemical substances cited in National Library of Medicine (NLM) databases, including the TOXNET system. | |
Record name | 3-Chloroallyl alcohol | |
Source | EPA DSSTox | |
URL | https://comptox.epa.gov/dashboard/DTXSID5041481 | |
Description | DSSTox provides a high quality public chemistry resource for supporting improved predictive toxicology. | |
Record name | 3-chloroprop-2-en-1-ol | |
Source | European Chemicals Agency (ECHA) | |
URL | https://echa.europa.eu/information-on-chemicals | |
Description | The European Chemicals Agency (ECHA) is an agency of the European Union which is the driving force among regulatory authorities in implementing the EU's groundbreaking chemicals legislation for the benefit of human health and the environment as well as for innovation and competitiveness. | |
Explanation | Use of the information, documents and data from the ECHA website is subject to the terms and conditions of this Legal Notice, and subject to other binding limitations provided for under applicable law, the information, documents and data made available on the ECHA website may be reproduced, distributed and/or used, totally or in part, for non-commercial purposes provided that ECHA is acknowledged as the source: "Source: European Chemicals Agency, http://echa.europa.eu/". Such acknowledgement must be included in each copy of the material. ECHA permits and encourages organisations and individuals to create links to the ECHA website under the following cumulative conditions: Links can only be made to webpages that provide a link to the Legal Notice page. | |
Record name | 3-CHLOROALLYL ALCOHOL | |
Source | FDA Global Substance Registration System (GSRS) | |
URL | https://gsrs.ncats.nih.gov/ginas/app/beta/substances/BP3J7M3WWA | |
Description | The FDA Global Substance Registration System (GSRS) enables the efficient and accurate exchange of information on what substances are in regulated products. Instead of relying on names, which vary across regulatory domains, countries, and regions, the GSRS knowledge base makes it possible for substances to be defined by standardized, scientific descriptions. | |
Explanation | Unless otherwise noted, the contents of the FDA website (www.fda.gov), both text and graphics, are not copyrighted. They are in the public domain and may be republished, reprinted and otherwise used freely by anyone without the need to obtain permission from FDA. Credit to the U.S. Food and Drug Administration as the source is appreciated but not required. | |
Retrosynthesis Analysis
AI-Powered Synthesis Planning: Our tool employs the Template_relevance Pistachio, Template_relevance Bkms_metabolic, Template_relevance Pistachio_ringbreaker, Template_relevance Reaxys, Template_relevance Reaxys_biocatalysis model, leveraging a vast database of chemical reactions to predict feasible synthetic routes.
One-Step Synthesis Focus: Specifically designed for one-step synthesis, it provides concise and direct routes for your target compounds, streamlining the synthesis process.
Accurate Predictions: Utilizing the extensive PISTACHIO, BKMS_METABOLIC, PISTACHIO_RINGBREAKER, REAXYS, REAXYS_BIOCATALYSIS database, our tool offers high-accuracy predictions, reflecting the latest in chemical research and data.
Strategy Settings
Precursor scoring | Relevance Heuristic |
---|---|
Min. plausibility | 0.01 |
Model | Template_relevance |
Template Set | Pistachio/Bkms_metabolic/Pistachio_ringbreaker/Reaxys/Reaxys_biocatalysis |
Top-N result to add to graph | 6 |
Feasible Synthetic Routes
A: 3-Chloroallyl alcohol is a major degradation product of the soil fumigant 1,3-dichloropropene (1,3-D). [, , , , , , ] 1,3-D is used to control nematodes in soil and its application leads to the formation of 3-CAA in groundwater. [, , ]
A: Plants readily absorb and metabolize 3-CAA. [, , ] It is further transformed into 3-chloro-1-propanol and 3-chloroacrylic acid. [, ] Eventually, these metabolites are incorporated into general plant constituents, including glucose, TCA acids, amino acids, and lipids. []
A: No, this compound has a short half-life in plants. Studies show it becomes undetectable after 96 hours in tomatoes. [] Similarly, 1,3-dichloropropene, its precursor, is not found after 72 hours. []
A: While 3-CAA itself degrades rapidly in plants, the long-term persistence of 1,3-dichloropropene and its impurities in soil is a concern. [] Specifically, 1,2,2-trichloropropane, an impurity in some 1,3-D formulations, was identified as having a higher risk of groundwater contamination. []
A: Hydrolysis of 1,3-dichloropropene produces this compound. [] This reaction appears independent of pH but is influenced by temperature, with a half-life ranging from 3 days at 30°C to 51 days at 10°C. [] Interestingly, the cis:trans ratio of the isomers remains consistent throughout the hydrolysis process. []
A: Yes, soils with a history of repeated 1,3-dichloropropene applications show enhanced degradation rates compared to soils with limited or no previous exposure. [] This suggests the potential development of microbial communities capable of degrading 1,3-D and its metabolites more efficiently. []
ANone: Various methods have been employed, including:
- Repacked soil columns: Used to simulate leaching behavior and study the compound's fate in soil layers. []
- Laboratory incubation systems: Allow for controlled conditions to assess degradation rates in different soil types. []
- Radiolabeling (¹⁴C): Facilitates tracking the fate and distribution of the compounds and their metabolites in plants and soil. [, , , ]
- Gas chromatography: Used for the detection and quantification of 1,3-dichloropropene, this compound, and other metabolites in plant tissues. [, ]
- High-performance liquid chromatography (HPLC): A technique used to identify and quantify 1,3-D and its metabolites, such as this compound, in various matrices. []
Disclaimer and Information on In-Vitro Research Products
Please be aware that all articles and product information presented on BenchChem are intended solely for informational purposes. The products available for purchase on BenchChem are specifically designed for in-vitro studies, which are conducted outside of living organisms. In-vitro studies, derived from the Latin term "in glass," involve experiments performed in controlled laboratory settings using cells or tissues. It is important to note that these products are not categorized as medicines or drugs, and they have not received approval from the FDA for the prevention, treatment, or cure of any medical condition, ailment, or disease. We must emphasize that any form of bodily introduction of these products into humans or animals is strictly prohibited by law. It is essential to adhere to these guidelines to ensure compliance with legal and ethical standards in research and experimentation.