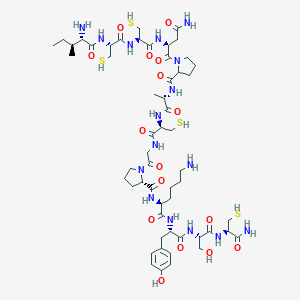
alpha-Conotoxin SI
Overview
Description
α-Conotoxin SI is a 13-residue peptide neurotoxin isolated from the venom of the marine cone snail Conus striatus. Its primary sequence is Ile-Cys-Cys-Asn-Pro-Ala-Cys-Gly-Pro-Lys-Tyr-Ser-Cys-NH2, stabilized by two disulfide bonds (Cys2-Cys7 and Cys3-Cys13) . Unlike other α-conotoxins, α-SI uniquely features a proline residue at position 9 instead of a positively charged amino acid (e.g., arginine in α-conotoxin GI), which critically influences its receptor specificity and binding dynamics . It selectively targets muscle-type nicotinic acetylcholine receptors (nAChRs) but lacks the ability to distinguish between the two agonist binding sites (α/γ and α/δ) on Torpedo californica receptors .
Preparation Methods
Synthetic Routes and Reaction Conditions: The synthesis of Conotoxin SI involves solid-phase peptide synthesis (SPPS), a method commonly used for the production of peptides. The process begins with the assembly of the linear peptide chain on a solid support, followed by the selective formation of disulfide bonds. The disulfide bonds are crucial for the peptide’s stability and biological activity. Various oxidative folding conditions are employed to ensure the correct formation of these bonds .
Industrial Production Methods: Industrial production of Conotoxin SI is still in its nascent stages, primarily due to the complexity of its synthesis and the need for precise control over disulfide bond formation. Advances in peptide synthesis technologies and optimization of folding conditions are expected to facilitate larger-scale production in the future .
Chemical Reactions Analysis
Types of Reactions: Conotoxin SI undergoes several types of chemical reactions, including:
Oxidation: Formation of disulfide bonds between cysteine residues.
Reduction: Breaking of disulfide bonds to yield free thiol groups.
Substitution: Modifications of amino acid residues to enhance stability or activity
Common Reagents and Conditions:
Oxidation: Typically achieved using reagents like iodine, thallium trifluoroacetate, or a sulfoxide/silyl mixture.
Reduction: Commonly performed using reducing agents such as dithiothreitol (DTT) or tris(2-carboxyethyl)phosphine (TCEP).
Substitution: Involves the use of various protecting groups and coupling reagents during SPPS
Major Products: The primary product of these reactions is the correctly folded Conotoxin SI with its native disulfide bond pattern. Misfolded isomers and partially oxidized intermediates are common by-products .
Scientific Research Applications
Neuropharmacological Studies
Alpha-conotoxin SI is instrumental in neuropharmacological studies aimed at understanding the role of nAChRs in various neurological conditions. Its selective inhibition properties make it a valuable tool for:
- Investigating Neuromuscular Transmission : By blocking specific nAChR subtypes, researchers can elucidate the mechanisms underlying neuromuscular diseases such as myasthenia gravis.
- Pain Management Research : Given the involvement of nAChRs in pain pathways, this compound is being explored for its potential analgesic properties, contributing to the development of new pain relief therapies .
Drug Discovery
The unique structure and function of this compound have made it a candidate for drug development:
- Pharmacological Probes : It serves as a model for designing new drugs targeting nAChRs, with implications for treating conditions like Alzheimer's disease and schizophrenia where cholinergic signaling is disrupted .
- Synthetic Analog Development : Researchers are creating synthetic analogs of this compound to enhance its pharmacokinetic properties and receptor selectivity. These modifications aim to improve stability and efficacy while reducing potential side effects .
Structural Studies
Recent studies have focused on the structural characterization of this compound, revealing insights into its binding affinity and specificity:
- A comparative analysis of this compound with other conotoxins highlighted its lower toxicity and unique binding characteristics, suggesting potential therapeutic advantages in clinical applications .
- Structural modifications have been explored to enhance its affinity for specific nAChR subtypes, leading to the development of more effective therapeutic agents .
Clinical Relevance
This compound's applications extend beyond basic research into potential clinical settings:
- Its role in modulating synaptic transmission has implications for treating neurodegenerative diseases and conditions characterized by altered cholinergic signaling.
- Ongoing studies aim to evaluate its effectiveness in preclinical models of pain and cognitive disorders, paving the way for future clinical trials .
Mechanism of Action
Conotoxin SI exerts its effects by binding to nicotinic acetylcholine receptors at the neuromuscular junction. This binding inhibits the normal function of these receptors, leading to a blockade of synaptic transmission. The peptide’s structure, stabilized by disulfide bonds, allows it to interact specifically with the receptor’s binding site, preventing the binding of acetylcholine and subsequent ion channel opening .
Comparison with Similar Compounds
Comparison with Similar α-Conotoxins
Sequence and Structural Differences
α-Conotoxin SI shares high homology with other α-conotoxins, such as GI, MI, and ImI, but diverges at key residues (Table 1):
Table 1: Sequence Comparison of α-Conotoxins
Conotoxin | Sequence (Key Residues Highlighted) | Key Structural Features |
---|---|---|
SI | I-C-C-N-P-A-C-G-P-K-Y-S-C | Pro9, Lys10, no Arg |
GI | E-C-C-N-P-A-C-R-G-Y-S-C | Arg9, Tyr10 |
MI | G-C-C-N-P-A-C-H-V-G-Y-C | His9, Val10 |
ImI | G-C-C-S-D-P-T-C-N-M-R-C | Arg7, Met10 |
The Pro9 in SI disrupts the conserved positive charge at this position, reducing its affinity for the α/γ site of muscle nAChRs compared to GI and MI . NMR studies confirm that SI adopts a backbone conformation similar to GI’s crystal structure but lacks the electrostatic interactions conferred by Arg9, explaining its distinct pharmacological profile .
Receptor Selectivity and Binding Affinity
α-Conotoxin SI exhibits broad specificity for muscle-type nAChRs but fails to discriminate between α/γ and α/δ agonist sites in Torpedo receptors, unlike GI and MI, which show 10–20-fold higher affinity for α/δ sites (Table 2) .
Table 2: Binding Affinity and Selectivity
Conotoxin | Target Receptor Subtype | IC50 (nM) | Site Selectivity (α/γ vs. α/δ) |
---|---|---|---|
SI | Muscle nAChR (Torpedo) | 20–50 | No selectivity |
GI | Muscle nAChR (Torpedo) | 1–5 | Prefers α/δ site |
MI | Muscle nAChR (Torpedo) | 2–8 | Prefers α/δ site |
ImI | Neuronal α7 nAChR | 200–500 | Targets homomeric α7 |
- Mechanistic Insights: The Arg9 in GI forms a salt bridge with the receptor’s γ-subunit, enhancing α/δ site selectivity. SI’s Pro9 abolishes this interaction, leading to non-selective binding . Mutagenesis studies show that replacing SI’s Pro9 with lysine ([P9K]SI) increases affinity for α/δ sites by 10–20-fold, mimicking GI’s behavior .
Pharmacological and Functional Differences
SI vs. GI/MI :
- SI vs. α-Conotoxin BuIA distinguishes neuronal α3β4 and α6/α3β4 nAChRs, a specificity absent in SI .
Biological Activity
Alpha-conotoxin SI is a peptide neurotoxin derived from the venom of cone snails, specifically from the species Conus striatus. This compound has garnered significant interest in pharmacology due to its selective antagonistic properties on nicotinic acetylcholine receptors (nAChRs). Understanding its biological activity is crucial for potential therapeutic applications, especially in treating conditions related to nAChR dysfunction.
This compound exhibits a distinct structure characterized by a conserved disulfide bond framework, which is essential for its biological activity. The peptide's interaction with nAChRs is mediated through competitive antagonism, where it binds to specific sites on the receptor, inhibiting acetylcholine's action.
Key Structural Features
- Disulfide Bonds : The presence of two disulfide bonds contributes to the stability and conformation necessary for receptor binding.
- Residue Variation : Variations in specific amino acid residues influence the affinity and selectivity of this compound for different nAChR subtypes.
Biological Activity
This compound has been shown to selectively inhibit various nAChR subtypes, with particular emphasis on its interaction with the alpha/delta interface of muscle-type nAChRs. Its potency varies significantly across different receptor types, highlighting its potential as a pharmacological probe.
Potency and Selectivity
- High Affinity : this compound displays a high affinity for the alpha/delta site on BC3H-1 receptors (IC50 values reported around 10 nM) but lower affinity for the alpha/gamma site compared to other conotoxins like GI and MI .
- Species-Specific Interactions : The toxin's binding characteristics can vary between species, showcasing a complex relationship with receptor subunit composition .
Comparative Analysis of Alpha-Conotoxins
To better understand the biological activity of this compound, a comparative analysis with other alpha-conotoxins is presented in the table below:
Conotoxin | IC50 (nM) | Preferred Binding Site | Toxicity (in vivo) | Selectivity |
---|---|---|---|---|
This compound | ~10 | Alpha/Delta on BC3H-1 receptors | Low | Selective for muscle-type nAChRs |
Alpha-Conotoxin GI | ~2 | Alpha/Gamma on Torpedo receptors | Moderate | High selectivity for Torpedo nAChRs |
Alpha-Conotoxin MI | ~5 | Alpha/Gamma on Torpedo receptors | High | High selectivity for muscle-type nAChRs |
Case Studies and Research Findings
- Mechanistic Insights : A study investigating the structural basis of this compound's action revealed that specific amino acid residues play critical roles in determining receptor interaction and selectivity. Mutagenesis studies indicated that changes at positions 9 and 10 significantly affect binding affinity at different receptor sites .
- Therapeutic Potential : Research has highlighted the potential use of this compound as a therapeutic agent in treating nicotine addiction and other disorders related to nAChR dysregulation. Its selective antagonism at specific receptor subtypes could lead to targeted therapies with fewer side effects compared to non-selective agents .
- Pharmacological Probes : Alpha-conotoxins, including SI, are being explored as pharmacological probes to elucidate the structure-function relationships of nAChRs. This research is pivotal in developing drugs that can modulate these receptors for therapeutic benefits .
Q & A
Basic Research Questions
Q. What experimental methods are used to determine the primary structure of alpha-Conotoxin SI?
The primary structure of this compound is typically resolved using Edman degradation for sequential amino acid identification and mass spectrometry (MALDI-TOF or ESI-MS) for molecular weight validation. These methods are complemented by cDNA cloning to confirm the peptide sequence derived from the venom of Conus striatus .
Q. How is this compound purified from cone snail venom, and how is its target specificity assessed?
Purification involves chromatographic techniques (e.g., reverse-phase HPLC and ion-exchange chromatography) to isolate the peptide from crude venom. Target specificity is evaluated via competitive binding assays using radiolabeled nAChR subunits (e.g., α3β4 or α7 subtypes) and electrophysiological recordings in heterologous expression systems (e.g., Xenopus oocytes) to measure receptor inhibition .
Q. What are the foundational steps for validating the interaction of this compound with nicotinic acetylcholine receptors (nAChRs)?
Key steps include:
- Receptor subtype expression in model systems (e.g., HEK293 cells).
- Dose-response curves to determine IC50 values.
- Schild analysis to confirm competitive antagonism.
- Cross-linking experiments or surface plasmon resonance (SPR) to quantify binding kinetics .
Advanced Research Questions
Q. How can researchers resolve contradictions in reported binding affinities of this compound across nAChR subtypes?
Discrepancies may arise from differences in receptor oligomerization, expression systems, or assay conditions. To address this:
- Standardize protocols using homomeric vs. heteromeric receptor configurations .
- Perform site-directed mutagenesis on key receptor residues (e.g., α3 Trp<sup>145</sup> or β4 Tyr<sup>121</sup>) to identify binding determinants.
- Combine molecular dynamics simulations (e.g., GROMACS) with alanine scanning mutagenesis of the toxin to map interaction interfaces .
Q. What methodologies are recommended for validating molecular docking models of this compound-nAChR interactions?
Computational models (e.g., GRAMM or GOLD docking) require experimental validation via:
- Cryo-EM or X-ray crystallography of toxin-receptor complexes.
- NMR spectroscopy to analyze toxin conformational changes upon binding.
- Functional assays with synthetic toxin analogues (e.g., Tyr<sup>12</sup>→Thr mutants) to test predicted binding residues .
Q. How can researchers design this compound analogues with enhanced selectivity for specific nAChR subtypes?
Strategies include:
- Alanine scanning to identify critical residues for receptor binding.
- Fluorescence labeling (e.g., FITC conjugation) for real-time binding visualization.
- Phage display libraries to screen for high-affinity mutants. Example: A fluorescent LtIA analogue (LtIA-FITC) was engineered to study α3β2 nAChR specificity via confocal microscopy .
Q. What approaches mitigate variability in functional assays measuring this compound activity?
To ensure reproducibility:
- Use internal controls (e.g., α-bungarotoxin for α7 nAChR inhibition).
- Standardize electrophysiological buffers (e.g., Ca<sup>2+</sup>/Mg<sup>2+</sup> concentrations).
- Apply statistical rigor (e.g., ANOVA with post-hoc tests) across biological replicates .
Q. Methodological Table: Key Techniques for this compound Research
Properties
IUPAC Name |
(2S)-1-[2-[[(2R)-2-[[(2S)-2-[[1-[(2S)-4-amino-2-[[(2R)-2-[[(2R)-2-[[(2S,3S)-2-amino-3-methylpentanoyl]amino]-3-sulfanylpropanoyl]amino]-3-sulfanylpropanoyl]amino]-4-oxobutanoyl]pyrrolidine-2-carbonyl]amino]propanoyl]amino]-3-sulfanylpropanoyl]amino]acetyl]-N-[(2S)-6-amino-1-[[(2S)-1-[[(2S)-1-[[(2R)-1-amino-1-oxo-3-sulfanylpropan-2-yl]amino]-3-hydroxy-1-oxopropan-2-yl]amino]-3-(4-hydroxyphenyl)-1-oxopropan-2-yl]amino]-1-oxohexan-2-yl]pyrrolidine-2-carboxamide | |
---|---|---|
Source | PubChem | |
URL | https://pubchem.ncbi.nlm.nih.gov | |
Description | Data deposited in or computed by PubChem | |
InChI |
InChI=1S/C55H88N16O16S4/c1-4-27(2)43(58)54(86)69-38(26-91)51(83)68-37(25-90)50(82)64-33(20-41(57)74)55(87)71-18-8-11-40(71)52(84)61-28(3)45(77)67-36(24-89)46(78)60-21-42(75)70-17-7-10-39(70)53(85)62-31(9-5-6-16-56)47(79)63-32(19-29-12-14-30(73)15-13-29)48(80)65-34(22-72)49(81)66-35(23-88)44(59)76/h12-15,27-28,31-40,43,72-73,88-91H,4-11,16-26,56,58H2,1-3H3,(H2,57,74)(H2,59,76)(H,60,78)(H,61,84)(H,62,85)(H,63,79)(H,64,82)(H,65,80)(H,66,81)(H,67,77)(H,68,83)(H,69,86)/t27-,28-,31-,32-,33-,34-,35-,36-,37-,38-,39-,40?,43-/m0/s1 | |
Source | PubChem | |
URL | https://pubchem.ncbi.nlm.nih.gov | |
Description | Data deposited in or computed by PubChem | |
InChI Key |
HZTKBXVGWKXCPU-DTTUQCIZSA-N | |
Source | PubChem | |
URL | https://pubchem.ncbi.nlm.nih.gov | |
Description | Data deposited in or computed by PubChem | |
Canonical SMILES |
CCC(C)C(C(=O)NC(CS)C(=O)NC(CS)C(=O)NC(CC(=O)N)C(=O)N1CCCC1C(=O)NC(C)C(=O)NC(CS)C(=O)NCC(=O)N2CCCC2C(=O)NC(CCCCN)C(=O)NC(CC3=CC=C(C=C3)O)C(=O)NC(CO)C(=O)NC(CS)C(=O)N)N | |
Source | PubChem | |
URL | https://pubchem.ncbi.nlm.nih.gov | |
Description | Data deposited in or computed by PubChem | |
Isomeric SMILES |
CC[C@H](C)[C@@H](C(=O)N[C@@H](CS)C(=O)N[C@@H](CS)C(=O)N[C@@H](CC(=O)N)C(=O)N1CCCC1C(=O)N[C@@H](C)C(=O)N[C@@H](CS)C(=O)NCC(=O)N2CCC[C@H]2C(=O)N[C@@H](CCCCN)C(=O)N[C@@H](CC3=CC=C(C=C3)O)C(=O)N[C@@H](CO)C(=O)N[C@@H](CS)C(=O)N)N | |
Source | PubChem | |
URL | https://pubchem.ncbi.nlm.nih.gov | |
Description | Data deposited in or computed by PubChem | |
Molecular Formula |
C55H88N16O16S4 | |
Source | PubChem | |
URL | https://pubchem.ncbi.nlm.nih.gov | |
Description | Data deposited in or computed by PubChem | |
Molecular Weight |
1357.7 g/mol | |
Source | PubChem | |
URL | https://pubchem.ncbi.nlm.nih.gov | |
Description | Data deposited in or computed by PubChem | |
CAS No. |
115797-06-3 | |
Record name | Conotoxin SI | |
Source | ChemIDplus | |
URL | https://pubchem.ncbi.nlm.nih.gov/substance/?source=chemidplus&sourceid=0115797063 | |
Description | ChemIDplus is a free, web search system that provides access to the structure and nomenclature authority files used for the identification of chemical substances cited in National Library of Medicine (NLM) databases, including the TOXNET system. | |
Retrosynthesis Analysis
AI-Powered Synthesis Planning: Our tool employs the Template_relevance Pistachio, Template_relevance Bkms_metabolic, Template_relevance Pistachio_ringbreaker, Template_relevance Reaxys, Template_relevance Reaxys_biocatalysis model, leveraging a vast database of chemical reactions to predict feasible synthetic routes.
One-Step Synthesis Focus: Specifically designed for one-step synthesis, it provides concise and direct routes for your target compounds, streamlining the synthesis process.
Accurate Predictions: Utilizing the extensive PISTACHIO, BKMS_METABOLIC, PISTACHIO_RINGBREAKER, REAXYS, REAXYS_BIOCATALYSIS database, our tool offers high-accuracy predictions, reflecting the latest in chemical research and data.
Strategy Settings
Precursor scoring | Relevance Heuristic |
---|---|
Min. plausibility | 0.01 |
Model | Template_relevance |
Template Set | Pistachio/Bkms_metabolic/Pistachio_ringbreaker/Reaxys/Reaxys_biocatalysis |
Top-N result to add to graph | 6 |
Feasible Synthetic Routes
Disclaimer and Information on In-Vitro Research Products
Please be aware that all articles and product information presented on BenchChem are intended solely for informational purposes. The products available for purchase on BenchChem are specifically designed for in-vitro studies, which are conducted outside of living organisms. In-vitro studies, derived from the Latin term "in glass," involve experiments performed in controlled laboratory settings using cells or tissues. It is important to note that these products are not categorized as medicines or drugs, and they have not received approval from the FDA for the prevention, treatment, or cure of any medical condition, ailment, or disease. We must emphasize that any form of bodily introduction of these products into humans or animals is strictly prohibited by law. It is essential to adhere to these guidelines to ensure compliance with legal and ethical standards in research and experimentation.